All published articles of this journal are available on ScienceDirect.
Urinary Biomarkers of Oxidative Stress in Atrial Fibrillation
Abstract
There is increasing evidence from molecular studies to support the role of inflammation and increased oxidative stress that produce structural and electrical atrial remodeling to produce Atrial Fbrillation (AF). Oxidative damage to cardiomyocytes yields chemical substances that are secreted in urine. These substances can serve as biomarkers that can be measured, potentially allowing clinicians to quantify oxidative damage to the heart.
1. INTRODUCTION
Atrial Fibrillation (AF) is a global epidemic, being the commonest cardiac arrhythmia observed in clinical practice affecting ≈5.2 million people in United States, 10 million people in China and 33 million people worldwide [1-4]. With an aging population, AF predisposes affected patients to a higher risk of stroke, heart failure and death, and a lower quality of life [5-7]. Furthermore, AF is also the most common serious cardiac arrhythmia in the surgical setting. The incidence of Postoperative Atrial Fbrillation (POAF) in patients with cardiac surgery is around 20 to 40% [8, 9]. Several risk factors of AF have been identified, including obesity, hypertension, diabetes and obstructive sleep apnea [10, 11]. Recently, inflammation and oxidative stress have been implicated in the pathogenesis of AF and its complications [12-18]. Accordingly, an imbalance between increased oxidative stress and decreased antioxidant capacity can be due to up-regulation of enzymes such as NADPH oxidase (NOX) and Myeloperoxidase (MPO), leading to the increased production of Reactive Oxygen Species (ROS) [19, 20]. The objective of this review article is to provide an overview of the relationships between oxidative stress, inflammation and AF, with a particular focus on quantifying urinary biomarkers to monitor occurrence and progression of AF.
2. PHYSIOLOGICAL RESPONSE TO OXIDATIVE STRESS
Cells undergo apoptosis or necrosis when they are under stress. Necrotic cells lose their cell integrity and leak their intracellular materials [21]. These materials serve as “danger signals” and human body respond to these substances by initiating inflammatory cascades, which involve cytokines such as interleukin (IL)-1, IL-6 and tumour necrosis factor alpha (TNF-alpha). Apoptotic cells are processed by Antigen Presenting Cells (APCs) such as macrophages, releasing cytokines to recruit other immune cells to resolve damage [22]. There are two different types of macrophages: M1 and M2 with distinct functions. M1 macrophages are recruited during the early stage of cell damage with a role to clear dead cells whilst M2 macrophages are recruited later to promote healing by releasing pro-angiogenic and fibrotic mediators [23].
ROS is produced from normal cellular respiration, exposure to radiation and contact with toxins [24]. The major sources of ROS in cardiac cells include the byproducts of endogenous and exogenous redox systems, such as NADPH oxidase, xanthine oxidase, the mitochondrial electron transport chain, myeloperoxidase (MPO), and uncoupled nitric oxide synthase [25, 26]. A moderate amount of ROS is required to maintain homeostasis and to mediate physiologic responses via different cellular signaling pathways. Moreover, human body is resistant to mild oxidative stress by employing a defense system which involves enzymes, metal ions and endogenous antioxidant [24]. However, over-expression of NOX and MPO contributes to increased production of ROS. High levels of ROS could react with multiple biomolecules, such as proteins, deoxyribonucleic acid, ribonucleic acid, and lipids, leading to damage of the phospholipid bilayer and the loss of enzyme function, breaking the DNA strands, thereby leading to DNA mutations, lipid peroxidation, and cellular death [24, 27]. This can lead to electrophysiological and structural modelling of the atria and therefore to AF [28]. Arachidonic acid is a major component in the phospholipid bilayer. Peroxidation of arachidonic acid yields isoprostanes and their byproducts are readily excreted in urine. Likewise, 8-hydroxy-2'-deoxyguanosine (8-OHdG) and urinary biopyrins can also be found in urine when the body has been exposed to oxidative stress [29].
3. BIOCHEMISTRY OF DIFFERENT URINARY MARKERS
Biomarkers referred to “biological markers” are defined as the indicators of normal biological processes or pathologic processes that can be precisely measured and evaluated. They can be based on blood, urine, cardiac or cerebral imaging [17, 30-37]. Compared with invasive measurements of specific biomarker, noninvasive measurements are more feasible. Moreover, lipids appear to be the most susceptible to the attack of ROS among the biological molecules [38, 39]. Therefore, the levels of lipid peroxidation products may be used as an important biomarker for the measurement of oxidative stress status. The measurement of F2-isoprostanes by methods utilizing mass spectrometry is currently the best available biomarker of lipid peroxidation [38, 40].
Isoprostanes are a group of prostaglandin-like compounds derived from the free radical–catalyzed peroxidation of arachidonic acid [41-43]. In addition, 8-iso-PGF2α is an isoform of F2- isoprostanes and it has recently been identified as a urinary marker of oxidative stress [44] and may be useful for predicting AF.
8-OHdG is an oxidized nucleoside produced as a result of oxidative DNA damage [38]. It is readily excreted in urine and its concentration in urine reflects the extent of DNA damage in the body [45].
Biopyrins are oxidative metabolites of bilirubin produced in response to oxidative stress. They are immediately excreted into urine and can indicate the intensity of oxidation.
4. PATHOPHYSIOLOGICAL RELATIONSHIP
4.1. Oxidative Stress and AF
ROS are constantly produced as part of normal cellular metabolic processes (such as cellular respiration, antibacterial defense) and external exposures (such ionizing radiation, smoking, and toxins) as discussed earlier [46, 47]. Increased oxidative stress can arise from higher rates of ROS generation compared to its removal rates [15, 17]. As a result of this imbalance, excessive ROS can lead to both structural and electrical remodeling of the atrium [13, 48, 49]. ROS can alter the structure and function of cellular components, such as phospholipids, proteins, and mitochondrial DNA, leading to abnormal cellular signaling [49] (Fig. 1).
Increasing evidence demonstrated that oxidative stress plays an important role in the pathogenesis of AF [50, 51]. A recent study by Sovari et al. [52] indicated that the high levels of ROS were associated with increasing age, heart failure, diabetes mellitus, coronary artery disease and obesity, all of which are risk factors of AF. Several hypotheses have been proposed to explain the association between increased ROS and AF. First, high levels of ROS can augment cytokine production from activated inflammatory cells, leading to tissue damage [38, 53]. Secondly, ROS can induce cardiac structural and electrical remodeling. For example, oxidative stress could promote atrial remodeling, by modulating calcium handling proteins or ion channels [24]. These can in turn produce a combination of conduction defects or repolarization abnormalities that can predispose to focal or reentrant activity [54-56].
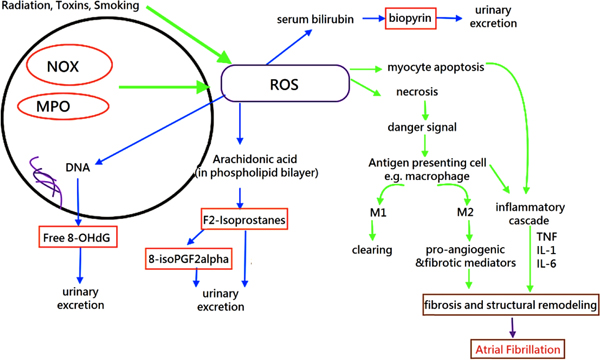
4.2. Inflammation, Increased Oxidative Stress and AF Pathogenesis
Experimental evidence suggests that inflammation plays a critical role in the pathogenesis of AF [10, 14-16, 57, 58]. The infiltration of inflammatory and immune cells, together with the expression of proteins, mediates the inflammatory response, cardiomyocyte necrosis, interstitial fibrosis and mitochondrial dysfunction, all of which are contributors to AF development and progression [48, 57, 59-62]. Previous studies have also identified the reciprocal relationship between angiotensin II (Ang II) and inflammation [49]. Ang II is a regulatory hormone released in response to changes in blood pressure. Experimental studies have demonstrated that Ang II increases the production of pro-inflammatory cytokines such as IL-6, IL-8, TNF-alpha, adhesion molecules and chemoattractant proteins [53, 63]. At the same time, inflammation increases Ang II production [64] and some inflammatory markers, such as CRP and TNF-alpha, increase Ang II receptor expression [65, 66]. This vicious cycle potentiates the development of AF. Indeed, increased levels of inflammatory markers, such as IL and C-Reactive Protein (CRP), are observed in AF patients [67, 68]. Inflammatory mediators mediate electrophysiological and structural remodeling, thereby increasing the vulnerability to AF [15, 69, 70].
5. CLINICAL STUDIES
A case-control study involving 305 patients with AF and 105 control patients demonstrated a positive relationship between inflammation and the development of AF [57]. More than that, higher concentrations of inflammation biomarkers vary with different stages of AF. IL-10, TNF-β, and NTpBNP concentrations were significantly higher in persistent or permanent AF compared to paroxysmal AF, whereas no significant differences in the concentrations of IL-8, Monocyte Chemoattractant Protein-1 (MCP-1), and Vascular Endothelial Growth Factor (VEGF) concentrations were observed in those three subtypes of AF after adjustment [57]. The Biomarkers of Oxidative Stress Study (BOSS) demonstrated that isoprostanes were the most sensitive biomarkers of endogenous oxidative stress compared with other biomarkers, such as oxidized lipid, protein, and DNA [39]. Urinary F2-isoprostanes detected by mass spectrometry are the most widely studied and generally accepted as the gold standard to measure lipid peroxidation and oxidative stress in vivo [44, 57, 71, 72]. Therefore, oxidative stress seems to have a direct relationship with AF and measuring urinary biomarkers is a potential tool to monitor the development and progression of AF.
5.1. Incident AF
Despite the fact that very few trials have studied the relationship between urinary biomarkers and the occurrence of postoperative AF, an ancillary study of the OPERA trial assessed the incidence of post-operative AF among 551 patients who received cardiac surgeries. This study revealed that urinary levels of F2-isoprostanes and isofuranes at the end of surgery were 20% and 50% higher in subjects who developed postoperative AF [51].
5.2. AF vs Non-AF: Cross-Sectional and Prospective Studies
Different studies have demonstrated that several biomarkers such as NOXs and MPO predict the risk of AF and morbidity and mortality in AF patients [50, 73]. A recent prospective cohort study found that 8-iso-PGF2α levels predict cardiovascular events and total mortality [47]. Several studies have assessed the predictive values of urinary biomarkers of oxidative stress in the context of AF, which are summarized in Table 1 [29, 51, 57, 74-76]. The study by Toyama et al. [29] affirmed that urinary 8-OHdG/creatinine levels were significantly increased in AF patients, and reduced when sinus rhythm restored. A prospective study contributed to the evidence in support of the potential pathological role of elevated oxidative stress in the setting of POAF [51]. In another prospective cohort study, F2-isoprostanes were significantly higher in patients who had recurrence of AF [74]. An additional study showed that urinary isoprostanes and NOX2 activity were significantly higher in patients with paroxysmal/persistent AF than in those with permanent AF and controls [76]. In contrast, an across-sectional study published by Li. et al. [57] showed no significant difference between the concentrations of F2-isoprostanes in the AF and those in the control groups. However, this study design did not take the effects of drugs, such as statins, anti-inflammatory agents, anti-diabetic medications, and angiotensin II blockers into consideration, that can potentially reduce the risk of AF [77]. These novel findings suggest that increased oxidative stress can be detected in the urine for risk stratification when confounding variables are well controlled. Despite most studies show a direct relationship between urinary biomarkers and AF, small sample sizes and the heterogeneity in the biomarkers make it difficult to draw definite conclusions. Therefore, future trials should include a larger group of candidates and unify the biomarkers of interest.
Author (Year) |
Study Design | Population (n) |
Endpoint | Biomarkers of Urine | Categories of AF | Measurement of Urine | Risk of the Marker of Urinary Oxidative Stress in Patients with AF | Categories of Results |
---|---|---|---|---|---|---|---|---|
Li (2010) |
CS | 305 patients with AF, and 150 control patients | NA | Urinary F2-isoprostanes | Lone/typical/paro-xysmal/persistent/ permanent AF | Using gas chromatography and mass spectroscopy. | Concentrations of Urinary F2-isoprostanes were not increased in AF over or in different subtypes of AF(p=0.50) | Not applicable |
Cangemi (2012) |
CS | 174 patients with AF, and 90 control patients | NA | Urinary isoprostanes | Paroxysmal/ persistent/ permanent AF | EIA assay method | Multivariable regression analyses: urinary isoprostanes were independently associated with the types of AF(paroxysmal/persistent VS permanent AF: β=-231, p=0.005) | Prognostic |
Ferro (2012) |
PS | 144 patients underwent electric cardio-version of nonvalvular persistent AF | AF recurrence | Urinary 8-iso-PGF2α | Non-valvular persistent AF (lasting≥7 days). | Using enzyme immunoassay method. | In unadjusted analysis, levels of urinary isoprostanes were significantly higher in patients with AF recurrence. Urinary isoprostanes levels were inversely correlated with serum vitamin E level (r=0.626, P=0.001). | Predictor of recurrence |
Toyama (2013) | – | – | – | – | – | – | – | |
Study 1 | CS | 173 patients with sinus rhythm and permanent AF | NA | Urinary 8-OHdG | Permanent AF | Enzyme-linked immunosorbent assay kit | Urinary 8-OHdG/Cr level was an independent factor that significantly correlated with the presence of permanent AF (β=0.36, p<0.001) | Predictor of incident AF |
Study 2 | CS | 36 cardiac surgery patients with persistent AF | NA | Urinary 8-OHdG and urinary biopyrrin | Persistent AF | EIA assay method | 8-OHdG/creatinine and biopyrrin/ creatinine levels at the chronic phase were significantly lower than those before cardioversion or catheter ablation (8.7±3.2 vs. 21.7±15.1 ng/mg, p.0001 and 1.7±1.1 vs. 3.0±1.9 mU/mg, p<0.0001). | Prognostic |
Pignatelli (2015) | PS | 1002 anticoagulated AF patients | All major CV events and death | Urinary 8-iso-PGF2a | Anticoagulated AF | A validate EIA assay method | 8-iso-PGF2a levels are predictive of CV events and total mortality. F2-IsoP complements risk factors in prediction of CV events. | Prognostic |
Wu (2015) |
PS | 551 cardiac surgery patients | Incident POAF | Urinary F2-isoprostanes, F3-isoprostanes, and isofurans | POAF of ≥30 seconds’ duration | Using gas chromatography and mass spectrometry | Multivariate-adjusted OR for urinary Isofurans and F3-isoprostanes were 1.95 (95%CI 1.05-3.62 p=0.01) and 2.10 (95%CI 1.04-2.25, p=0.04), respectively. | Predictor of incident AF |
5.3. Therapeutic Outcomes
Many studies support the notion that increased oxidative stress links to the development of AF. Accordingly, reduction in oxidative stress and neutralizing ROS are the keys to reduce the incidence of AF. In an experimental model of AF induced by atrial rapid pacing, enhanced oxidative stress was demonstrated and the vulnerability of AF was reduced by antioxidant treatment [19]. Pre-clinical and clinical studies have also demonstrated an association between biomarkers of oxidative stress and AF [19, 20] and that lower levels of antioxidants increased the occurrence or recurrence of AF in patients undergoing cardiac surgery [50, 74]. Indeed, the impact of antioxidants has attracted a number of interventional trials on the effect of antioxidants on postoperative AF. A meta-analysis reviewed 15 studies to examine if antioxidants could stop or retard the incidence of AF. Despite these studies have small sample size (ranging from 10 to 104), they consistently showed that antioxidants (vitamin C and N-acetylcysteine) reduced the incidence of AF after cardiac procedure [78].
5.4. Prognostic Implications
The measurement of oxidative stress biomarkers using the products of non-enzymatic reactions between biological molecules and ROS has been an area of intense study [46, 79]. In clinical practice, it is difficult to directly detect ROS in vivo, since they have short lifetimes and reliable methods for quantifying oxidative stress are lacking [80, 81]. On the other hand, urinary biomarkers of oxidative stress are useful indices of redox balance, and have advantages over traditional serum biomarkers because they can be obtained non-invasively and have a low organic/metal content ratio [46]. Moreover, urinary biomarkers can reflect redox balance over a prolonged period, compared to shorter half-lives of Reactive Oxygen Species (ROS) detected in serum biomarkers [46, 79]. Consequently, such urinary biomarkers can be a key indicator for predicting the occurrence or recurrence of AF and cardiovascular events from AF [29, 51, 57, 74-76]. Among the different urinary biomarkers, F2-isoprostanes are useful markers for assessing oxidant injury. Quantifications of F2-isoprostanes in urine varied in different methods. In general, liquid chromatography tandem-mass spectrometry (LC/MS/MS) is a sensitive and specific analytic method by using a solid phase extraction method. A 24-hour urine sample (2ml) of the patients was collected and analyzed, which facilitated us in clinical practice [82].
Studies suggest the cause of AF is inflammation related. Additionally, these findings may potentially have therapeutic implications, as anti-inflammatory therapy has the potential to reduce the occurrence and recurrence of AF [83, 84].
CONCLUSION
Recent studies support the idea that oxidative stress and inflammation are main contributors to AF. There is accumulating experimental evidence to suggest that urinary biomarkers of oxidative stress, such as F2-IsoPs, can be used for the prediction of AF. F2-isoprostanes and 8-OHdG are currently the recommended biomarkers for monitoring oxidative status, and can be used in large-scale clinical studies.
CONSENT FOR PUBLICATION
Not applicable.
CONFLICT OF INTEREST
The authors declare no conflict of interest, financial or otherwise.
ACKNOWLEDGEMENTS
This work was supported by grants (30900618, 81270245, and 81570298 to T.L.) from the National Natural Science Foundation of China, and Tianjin Natural Science Foundation