All published articles of this journal are available on ScienceDirect.
Transfer Proteins, Lipoprotein Remodeling, and Antioxidant Action on Pregnant Women: Comparison from the First to the Third Trimester of Gestation
Abstract
Introduction:
During pregnancy, maternal lipid metabolism undergoes several transformations originated by hormonal changes. There are two distinct phases: initial and late, finally driven to hypertriglyceridemia. Maternal hyperlipidemia, especially hypertriglyceridemia, is associated with an increased hepatic very-low-density lipoprotein (VLDL) synthesis stimulated by high estrogen levels during gestation.
Objectives:
This study aimed to evaluate the action of possible lipoproteins remodeling modulators, such as phospholipid and cholesteryl-ester transfer proteins (PLTP and CETP), paraoxonase-1 (PON-1), and apolipoproteins (apo), during gestation.
Methods:
An observational prospective cohort study composed of 40 pregnant women was conducted. Blood samples were collected in two moments: at the first and third trimesters of gestation, followed by the biochemical determination of apo A-I, apo B, lipid profile, PON-1, PLTP and CETP activities, and HDL particle size.
Results:
The majority of pregnant women in the third trimester showed dyslipidemia, mainly hypertriglyceridemia. In the third trimester of gestation, we observed an increase in CETP activity (70.5 ± 9.6 pmol/µL/h; p <0.001) and TG/HDL-C ratio (2.7 [2.0-3.8]), but on the other hand, PON-1 activity (65.4 [46.5-105] nMol of p-nitrophenol/min/mL) decreased. High-density level lipoprotein (HDL) particle size and PLTP activity did not differ in the two studied moments. The serum triglyceride concentration and CETP activity showed a positive linear correlation in the first trimester of gestation (r2=0.65, p<0.001).
Conclusion:
Data suggest that the CETP activity, antioxidant profile, and low-density lipoprotein (LDL) remodeling are modified by physiological metabolic changes during an uncomplicated gestational period.
1. INTRODUCTION
During pregnancy, maternal lipid metabolism undergoes several transformations originated by hormonal changes [1, 2]. There are two distinct phases: an initial phase characterized by an accumulation of fatty acids, resulting from the deposition of triglycerides (TG) in maternal adipose tissue, and the late phase characterized by the accelerated catabolism of adipose tissue, with the increased availability of fatty acids and glycerol in the circulation, generating hepatic very-low-density lipoprotein (VLDL) secretion and hypertriglyceridemia [3]. Maternal hyperlipidemia, especially hypertriglyceridemia, may be associated with the increased hepatic synthesis of VLDL originated by a high concentration of estrogen during gestation and the reduction of TG-rich lipoproteins catabolism due to low activity of lipoprotein lipase (LPL) and hepatic lipase (LH) [4-6].
Hypertriglyceridemia during pregnancy is responsible for modifying different pathways of lipid and lipoprotein metabolism. Besides increased cholesteryl ester transfer protein (CETP) activity, some studies have shown alterations in the composition and size of lipoproteins, such as higher TG content and the formation of smaller and denser particles [7, 8]. The accumulation of small and dense low-density lipoprotein (LDL) during gestation increases the risk of endothelial damage and oxidative stress [2].
The evaluation of high-density lipoprotein (HDL) functionality, together with serum HDL-cholesterol (HDL-C), is essential for a cardiovascular risk assessment [9, 10]. These protective characteristics can be altered in the presence of pro-inflammatory conditions and diverse modifications, such as oxidative changes [11]. A considerable cardiovascular risk may exist even with conditions, such as a high HDL cholesterol concentration, and therefore, the analysis of HDL metabolism biomarkers, such as apolipoprotein (apo) A-I, and HDL particle size, must be performed [11].
Maternal hyperlipidemia may be associated with the development of maternal-fetal complications (preterm birth, preeclampsia, vascular complications) and the development of cardiovascular diseases in the long term [3, 4]. The evolution of pregnancy represents an early opportunity to identify the presence of cardiovascular risk biomarkers [12]. Studies suggest that gestation constitutes a “stress test” for the metabolic pathways of carbohydrates and lipids. Besides causing inflammatory biomarkers hyperregulation, it also changes vascular function. Thus, this period may be an adequate time for the long-term prediction of hemodynamic complications [13-15].
The purpose of the present study was to evaluate the lipoprotein modulators, lipoprotein particle quality, and functionality during uncomplicated pregnancy.
2. MATERIALS AND METHODS
2.1. Patients
An observational prospective cohort study, comprised of 40 pregnant women selected by convenience, aged between 18 and 38 years, attended at the Climério de Oliveira Hospital Maternity, Federal University of Bahia (UFBA), Brazil, underwent prenatal follow-up between August 2014 and July 2015. The pregnant women were informed about the study and signed the Free Prior Informed Consent Form (FPIC). A socioeconomic and epidemiological questionnaire was used to address the information about cardiovascular risk factors, such as a sedentary lifestyle, alcohol consumption, smoking, a family history of cardiovascular diseases, use of medicines, and hypertension. Blood samples were obtained after 12-hour fasting on two occasions: in the first and third trimester of gestation. This study was approved by the Climério de Oliveira Hospital Maternity Research Ethics Committee (UFBA) (CEP/MCO/UFBA), according to the registration and additive resolution nº 103/2005 and nº029/2014, respectively.
Inclusion criteria were pregnant women in the first trimester of gestation, followed under prenatal care at the Climério de Oliveira Hospital Maternity (UFBA), with a low-risk pregnancy, and signing the Free Prior Informed Consent Form.
Exclusion criteria were women presenting with hepatic dysfunction, with alanine aminotransferase (ALT) and aspartate aminotransferase (AST) concentrations higher than twice the upper reference limit; renal dysfunction (serum creatinine >2.0 mg/dL), thyroid dysfunction, and diabetes mellitus.
To find a predictive number of women with uncomplicated pregnancies, following all restrictions applied to human ethics, our calculated population sample size was 40 cases. The blood samples were obtained on two gestational occasions, at the first and third trimesters, reaching 80 samplings. The sample size calculation conditioned an 80% (1-beta) predictive minimum statistical power to find 4 unit differences in paraoxonase activity at a maximum of 10% variation between pre and post-tests. The sample size calculations were performed with WINPEPI for Windows, version 11.48 (Joe Abramson, PEPI; programs for epidemiologists), and the predictive power was estimated with StatMate 2 for Windows, version 2.0 (GraphPad Software, inc, 1992-2004).
2.2. Biochemical Determinations
Blood samples were obtained after 12 hours of fasting. The biochemical determinations were performed by spectrophotometry, kinetic, and colorimetric methods using the automated equipment METROLAB 2300 (Wiener Lab, Argentina) in plasma and/or serum samples. The LDL-cholesterol levels were estimated by the Friedewald formula [16], and VLDL-cholesterol was calculated as triglycerides level divided by 5.
2.3. PLTP and CETP Activity
Plasma PLTP and CETP activities were determined using a fluorescence-based assay kit (BioVision Inc. USA). Plasma PLTP and CETP activities were expressed by picomoles of neutral lipid transferred per microliter of plasma per hour (pmol/µL/h). To determine the activities of the transfer proteins, we used an EnVision®, Multilabel Reader 2104 (PerkinElmer, USA).
2.4. PON-1 Activity
The paraoxonase 1 (PON-1) activity was measured according to Charlton-Menys et al. [17], Senti et al. [18], and Oliveira et al. [19] using 140 µL of 0.1 M Tris-HCl (pH 8.5, containing 2mmoL/L CaCl2, paraoxon 1.1 mmoL/L, Sigma Chemical Co.) and 7µL of serum. Samples were pipetted by duplicate to run into a 96-well ELISA plate. Readings were made at 405nm, 37 °C, using a Microplate Reader (Benchmark, Bio-Rad, USA). For activity calculation, six readings were made at intervals of one minute each, and results were calculated by multiplying the obtained factor by the average absorbance variation (PON-1 activity = Factor x Δ Absorbance/minute).
2.5. Apo B and Apo A-I Concentration
The apo B and apo A-I levels were measured by immunonephelometry using an automated analyzer IMMAGE® (Beckman Coulter, USA), by the measurement of light scattered or reflected when radiant energy passed through a solution and encountered a molecule on elastic collision. The resultant light was detected in a different path than the directly transmitted light.
2.6. HDL Particle Size
The HDL particle size diameters were measured by laser light scattering (LLS) using a Zetasizer Nano ZSP (Malvern, United Kingdom) as previously described by our group, Lima and Maranhão [20] and Santos et al. [21]. EDTA plasma was obtained by tube centrifugation for 15 min at 1250g. For the isolation of HDL, 500uL of precipitating solution of polyethylene glycol (PEG) 8000 (400 g/L), in 0.2 mol/L glycine buffer adjusted to pH 10, in 500uL of EDTA plasma. Samples were vigorously mixed for 30 seconds and then centrifuged for 10 minutes at 1800g for apo B-containing lipoprotein precipitation. A 500uL supernatant was added to 1.5 mL of NaCl (10 mmol/L) and passed through a 0.22 μm filter (Millipore Products Division) to exclude undesirable particles and dispensed into the equipment cuvette. The scattered light was collected at an angle of 90˚ by a photon-counting photomultiplier tube directed to an equipment correlator. The sample results were expressed as the mean particle size, a harmonic intensity-averaged particle diameter. All experiments were performed at 22˚C, and the resultant data were expressed employing ten runs of 1 minute each.
2.7. Estimation of LDL Particle Size
The LDL particle size was estimated by using the TG/HDL-C ratio. A ratio higher than 2.0 was considered indicative of sdLDL (≤25.5nm) [22].
2.8. Statistical Analyses
Descriptive and inferential statistical analysis was performed. In the first step, centrality and dispersion measurements were calculated, and afterward, the D´Agostino test was used to test the type of data distribution around the mean. According to the type of data distribution, the tests for inferential analysis were chosen, such as paired t-test or Wilcoxon one-way test, by prior hypothesis postulation. The paired t-test was used to analyze the following parameters: glucose, urea, ALT, lipid profile, apolipoproteins, HDL-particle size, PLTP, and CETP activity, and the risk ratios (LDL-C/apo B, HDL-C/apo A-I, and apo B/apo A-I). The Wilcoxon test was used to analyze PON-1 activity, TG/HDL-C ratio, creatinine, AST, CRP, hematocrit, and hemoglobin. The Pearson coefficient was used to calculate the linear correlation between plasmatic triglyceride concentration and CETP activity in the first and third trimesters of gestation. We also performed an association analysis (Fisher test) to evaluate the differences between proportions of the main factors and risk biomarkers for coronary artery disease (CAD) and the main outcome considered, the development of hypertriglyceridemia.
For outlier detection, Grubb´s test was employed. All tested data were considered significant when the critical value (p) was under 0.05 to a confidence interval of 0.95. The statistical analyses were performed at GraphPad Prism version 5.01 (USA) statistical packages.
3. RESULTS
The general characteristics and socioeconomic descriptive data from the study participants are shown in Table 1. Fig. (1) shows the observed frequency of the main risk factors for CAD development before becoming pregnant.
Characteristics | |
---|---|
Sample size (n) Gestational age: First trimester Third trimester |
40 10 ± 2 32 ± 3 |
Age (years) | 28 ± 7 |
Civil status: Married Single |
23 (57.5%) 17 (42.5%) |
Ethnicity: White Black Mixed |
3 (7.5%) 18 (45.0%) 19 (47.5%) |
Number of gestations: 1 2 3 |
26 (65.0%) 13 (32.5%) 1 (2.5%) |
Education: Basic education High school Higher education |
2 (5.0%) 31 (77.5%) 7 (17.5%) |
Monthly income: Family Dependents 1 Basic salary >1 Basic salary |
20 (50%) 16 (40%) 4 (10%) |
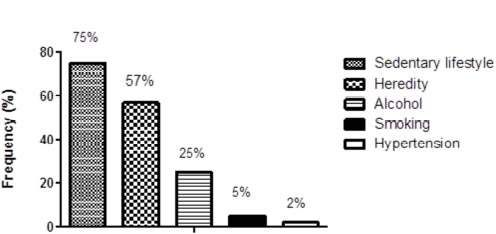
Variables | 1° Trimester | 3° Trimester | P |
---|---|---|---|
Glucose (mg/dL) a | 83 ± 8 | 84 ± 8.0 | Ns |
Urea (mg/dL)a | 13.3 ± 4.4 | 15.6 ± 4.1 | ** |
Creatinine(mg/dL)b | 0.7 (0.6-0.7) | 0.7 (0.5-0.8) | Ns |
AST (U/L)b | 16 (13-21) | 16 (13-20) | Ns |
ALT (U/L)a | 14.6 ± 5.2 | 10.9 ± 3.8 | *** |
CRP (mg/dL)b | 5.5 (3.2-13.5) | 9.6 (7.0-14.5) | ** |
Hb (mg/dL)b | 12 (11.7-12.6) | 11.7 (11.1-12) | *** |
Htc (%)b | 35.2 (34.4-36.1) | 34.6 (34-35.9) | *** |
Table 2 shows the biochemical and hematological parameters in the first and third trimesters of gestation, performed to evaluate the pregnant women’s health status. The levels of urea and CRP increased (p<0.001), while ALT, hemoglobin, and hematocrit decreased (p<0.0001) during the gestation.
Table 3 shows the lipid profile and the serum concentration of apolipoproteins in the first and third trimesters of gestation. Data show the development of dyslipidemia in the first and third trimesters, represented by increased LDL-C and triglycerides levels (p<0.0001). There was also a significant increase in the number of antiatherogenic and atherogenic particles, addressed by the apolipoprotein values (p<0.0001).
Lipid Profile | 1 ° Trimester | 3° Trimester | P | Reference Value* |
---|---|---|---|---|
Total cholesterol (mg/dL) | 164 ± 26 | 214 ± 43 | *** | < 190 |
Triglycerides (mg/dL) | 95 ± 32 | 180 ± 70 | *** | < 150 |
HDL-C (mg/dL) | 59 ± 9 | 59 ± 10 | Ns | > 40 |
LDL-C (mg/dL) | 86 ± 22 | 118 ± 37 | *** | < 130 |
VLDL-C (mg/dL) | 19 ± 6 | 36 ± 14 | *** | < 30 |
Apo A-I (mg/dL) | 190.3 ± 24.1 | 220.6 ± 35.5 | *** | 100 a 200 |
Apo B (mg/dL) | 110.7 ± 34.3 | 149.5 ± 47.8 | *** | 50 to 155 |
Risk Ratio | 1° Trimester | 3° Trimester | P |
---|---|---|---|
apo B/apo A-I | 0.58 ± 0.2 | 0.68 ± 0.2 | * |
HDL-C/apo A-I | 0.29 ± 0.02 | 0.25 ± 0.04 | *** |
LDL-C/apo B | 0.78 ± 0.13 | 0.83 ± 0.21 | ns |
Table 4 shows the calculated cardiovascular risk ratios used to estimate lipoprotein remodeling and composition and the number of circulating particles as suggestive markers of cardiovascular risk. Apo B/apo A-I ratio increased (p<0.01), and HDL-C/apo A-I decreased (p<0.0001) in the third trimester of gestation.
As shown in Fig. (2), the CETP activity significantly increased in the first and third trimesters of gestation (56.6±5.4 to 70.5±9.6 pmol/µL/h, p<0.0001). On the other hand, the PLTP activity did not show significant differences between the evaluated periods (28.9 ± 6.8 and 27.2 ± 9.1 pmol/µL/h, respectively).
Fig. (3) shows the linear correlation analysis between serum TG and CETP activity in the first (r2 = 0.70, Pearson correlation test, p<0.0001) and third trimesters of gestation (r2 = 0.33, Pearson correlation test, p=0.0209).
As shown in Fig. (4), there were no significant differences between HDL particle size in the first and third trimesters of gestation (11.78±1.2 and 11.8±1.0 nm).
To evaluate the mean size of circulating LDL particles, the TG/HDL-C ratio was used. A value above 2.0 is an indicator of small and dense LDL particle predominance [22]. TG/HDL-C ratio in the first (1.3; 1.2-2.0) and third (2.7; 2.0-3.8) trimesters of gestation shows significant differences (p<0.0001) (Fig. 5).
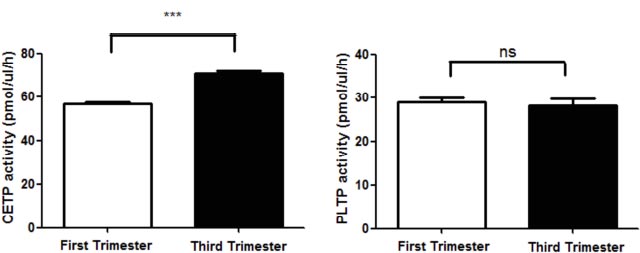
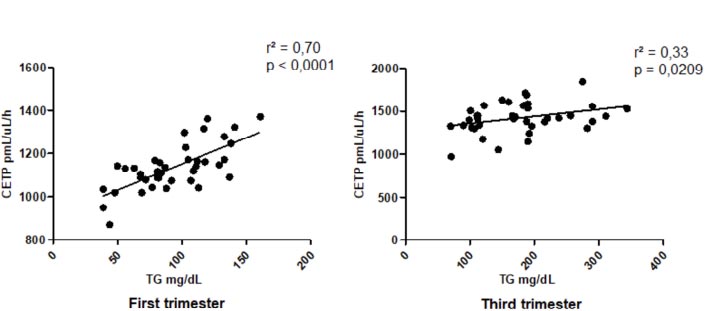
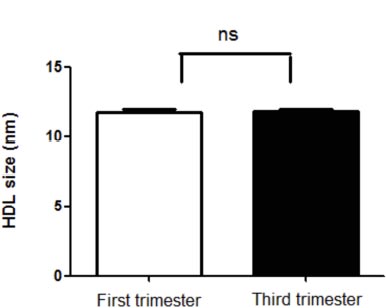
Fig. (6) shows the PON-1 antioxidant activity. There was a significant decrease in PON-1 activity from the third trimester of gestation (88.3 [61.3 -114.5] and 65.4 [46.5-105] nMol of p-nitrophenol/min/mL, p=0.0037).
4. DISCUSSION
Here, we evaluated the health status of pregnant women through the analyses of renal, hepatic, and inflammatory profiles, glucose, hematocrit, and hemoglobin levels. Among the findings, although some parameters differed significantly before and after, all results were inside the reference values. Most women start the gestational period healthily, but some may have a silent kidney or liver disease, subclinical, without adverse health effects [23, 24].
Importantly, in our study, the lipid profile of pregnant women shows marked alterations. According to Geraghty et al. [25], lipid metabolism during pregnancy is completely altered. By the final of gestation, all lipid biomarkers, such as lipoprotein particles carrying cholesterol and triglyceride, may increase. Lipids are essential for fetal development, as they act
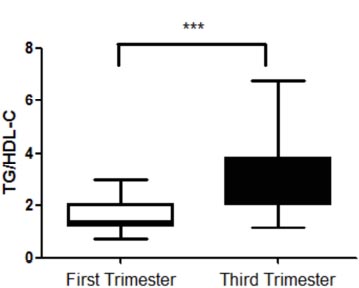
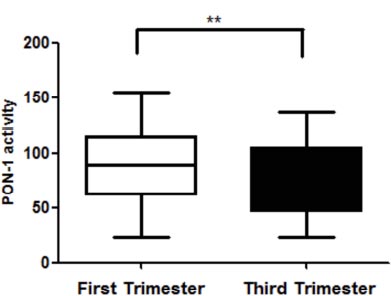
as a source of energy and as constituents of the cell membrane. Cholesterol and phospholipids increase moderately, and triglycerides increase sharply [26]. Hypertriglyceridemia is one of the main alterations in the lipid profile noticed at the end of gestation. However, it is linked to VLDL content modification, which also modifies LDL and HDL structures by fulfilling it with higher triglyceride content [27].
In the present study, the total cholesterol, lipoprotein cholesterol fractions, and triglycerides results were significantly increased during the gestational trimesters, except for HDL-C, which remained unaltered. According to Emet et al. [3], LDL-C, triglycerides, and HDL-C were significantly higher from the third trimester of gestation. Other studies have shown that the lipid profile becomes more atherogenic at the end of gestation [26, 28-30]. Vrijkotte et al. [31] found that hypertriglyceridemia in pregnancy is associated with maternal-fetal complications. It can compromise endothelial function and contribute to the development of atherosclerotic vascular disease, preeclampsia, and oxidative stress promotion [32, 33].
We noted that apo A-I and apo B increase from the third trimester of gestation. In the same way, the apo B/apo A-I ratio showed the same profile during this period. The study by Piechota and Staszewski [34] shows that apo A-I and apo B increased in the second and third trimesters, in which apo A-I increased by 32% and apo B by 56%. Belo et al. [35] conducted a longitudinal study to evaluate apolipoprotein concentration during the gestational period. They observed an apo B increasing from the first to the third trimesters of gestation, while apo A-I showed a slight reduction in the third trimester. Rymer et al. [36] concluded that in pregnant women, apo A-I and B concentrations were higher than those of non-pregnant women. The apo B/apo A-I ratio at the third trimester of gestation reflects the balance between two distinct processes: cholesterol transport to extrahepatic tissues and reverse transport to the liver. The increase of the apo B/apo A-I ratio, indicating the higher proportion of atherogenic lipoproteins in the plasma, is an index that reflects the dyslipidemia progression and endothelial dysfunction [37, 38].
Other cardiovascular risk ratios were evaluated in our study, such as HDL-C/apo A-I and LDL-C/apo B, which reflected lipoprotein remodeling, composition, and particle number [37]. The significantly reduced HDL-C/apo A-I ratio from the third trimester of gestation is suggestive of an HDL particle number increase with lower cholesterol content, suggesting an alteration in HDL particle composition and size. The LDL-C/apo B ratio did not change significantly during gestation, perhaps because the increase in cholesterol content of LDL is proportional to the increase in the apo B-containing particle number. A study by Tani et al. [39] showed that values ≤1.2 for the LDL-C/apo B ratio are suggestive of small and denser LDL particle predominance.
In our study, the CETP activity was different between the first and third trimesters of gestation, showing more frequent high activity at the end of gestation. The CETP activity influences the esterified cholesterol concentration and its distribution between lipoprotein classes, altering the lipoprotein composition, and thus influencing the lipoprotein profile atherogenicity [27, 40, 41]. In addition, the CETP increased activity plays a decisive role in the formation of small and denser LDL [42]. Patients with dyslipidemias, such as hypertriglyceridemia and hypercholesterolemia, with diabetes, obesity, or venous thrombosis have increased esterified cholesterol transfer from HDL to apo B-containing lipoproteins, as a result of an increased CETP activity, which contributes to a rise in the atherosclerosis progression and cardiovascular risk [27, 43]. Zhang et al. [44] have shown that endogenous estrogen can affect the CETP concentration in pregnant women´s plasma. It is known that in addition to hormonal status and metabolic conditions, lifestyle habits, such as diet and physical activity, can also influence CETP activity [45].
In the present study, a positive correlation between serum triglyceride and CETP activity in the first and third trimesters of gestation was found. The CETP activity did not increase proportionally as triglycerides from the third trimester, probably due to CETP enzymatic catalytic saturation, which may have masked the expected increase in CETP activity. Another significant finding was that CETP has a higher affinity for esterified cholesterol substrate than triglycerides as a function of the steric impediment since triglyceride molecule is approximately 1.5 times greater than esterified cholesterol [46]
In our study, the PLTP activity did not change significantly during pregnancy. PLTP contributes to HDL metabolism because it is associated with lipoprotein remodeling and reverse cholesterol transport [47]. The increase in PLTP activity leads to increased cholesterol efflux from the endothelial cell membranes to HDL, permitting the formation of larger and anti-inflammatory HDL particles [47]. According to Jiang et al. [48], the adipose tissue produces modulators that can interfere with the performance of PLTP activity. Furthermore, it is noteworthy that apo E plays an essential role in PLTP regulation, as an activator or just increasing its activity [49].
When evaluating the lipoprotein remodeling, there was no significant difference in HDL particle size compared to the first and third trimesters of gestation. Several HDL subpopulations show different qualitative properties that directly affect its activity [50]. HDL particles are quite heterogeneous structurally and functionally [51, 52]. Among the HDL subpopulations, the larger lipoprotein particles (the cholesterol-riches) are inversely related to cardiovascular risk, and the smaller particles (probably inefficient to remove cholesterol) show a cardiovascular-risk positive correlation [53-55].
LDL particles are also heterogeneous and have different physical and chemical characteristics [2]. In the present study, the LDL particle size was estimated by the TG/HDL-C ratio. From the third trimester of gestation, the values suggest a predominance of smaller, denser, more atherogenic particles. A study conducted by Jayanta et al. [56] showed that in the group of uncomplicated and non-hypertensive pregnant women, the TG/HDL-C ratio value from the third trimester of gestation was 3.6 higher than that found in our study. Meyer et al. [7] showed that in pregnant women, from the second and third trimesters, the proportion of small and dense LDL particles increased when compared to women in the first trimester of gestation and the postpartum period. This proportion was higher in obese pregnant women (40.7%) than those with normal weight (21.9%). Zeljkovic et al. [57], in a longitudinal study, concluded that LDL particle size reduced, but the proportion of small and dense LDL increased during gestation. The increase in the proportion of small and dense LDL particles is indicative of an atherogenic phenotype, which has been associated with the development of hepatic steatosis and has also been observed in women with gestational diabetes and preeclampsia [32, 58, 59].
Regarding the oxidative profile during pregnancy, the present study showed a decrease in PON-1 activity in the third trimester. A significant PON-1 activity decrease was observed in previously performed studies [60-62]. Stefanovic et al. [61] showed, in a longitudinal study, that a significant PON-1 activity decreased in the third trimester (32 weeks) of gestation. In addition, the lipid profile became more atherogenic. Similar results were found in the study conducted by Ferre et al. [60], where the PON-1 activity decreased in different moments (145.8 U/L at 8-20 weeks; 111.1 U/L at 32 weeks, and 100.4 U/L during labor). Ferretti et al. [63] evaluated the relationship between obesity and oxidative stress in pregnancy. Their data showed a decrease in PON-1 plasma activity in obese pregnant women, indicating that mothers and newborns are susceptible to greater tissue oxidative damage. Changes in lipid profile, body mass index, hormonal profile, and oxidative stress lead to PON-1 activity changes, mainly at the end of gestation. This process represents a decrease in antioxidant capacity and may be an additional reason for a cardiovascular risk increase in pregnant women [61]. Also, our findings from a previously published systematic review, an animal model [64], showed that an offspring of parents, mother, and father, exposed to a high-fat diet exhibited a variety of up and down gene expressions, influencing changes in the status of glycemia, insulinemia, pancreatic islet tissues transcriptome, levels of triglycerides, and lipogenic genes expression.
CONCLUSION
The development of dyslipidemia, mainly hypertriglyceridemia, during the gestational period associated with lipoproteins' structural and functional changes, such as CETP activity, increased apo B/apo A-I ratio, and decreased HDL antioxidant capacity, argues for the presence of an atherogenic lipid profile, with less LDL protection. Although the HDL particle remodeling does not change, the predomination of small and denser LDL particles at the end of gestation points to important lipoproteins' structure and function changes. The observed lipoprotein modifications in women with uncomplicated pregnancy alert for close monitoring of metabolic predisposing conditions that can aggravate cardiovascular risk.
LIST OF ABBREVIATIONS
(VLDL) | = Very-low-density Lipoprotein |
CETP | = Cholesteryl-ester Transfer Proteins |
(PON-1) | = Paraoxonase-1 |
(HDL) | = High-density Level Lipoprotein |
(LDL) | = Low-density Lipoprotein |
(ALT) | = Alanine Aminotransferase |
(AST) | = Aspartate Aminotransferase |
ETHICS APPROVAL AND CONSENT TO PARTICIPATE
This study was approved by the Climério de Oliveira Hospital Maternity Research Ethics Committee (UFBA) (CEP/MCO/UFBA), according to the registration and additive resolution no. 103/2005 and no. 029/2014, respectively.
HUMAN AND ANIMAL RIGHTS
No animals were used in this research. All procedures performed in studies involving human participants were in accordance with the ethical standards of institutional and/or research committee and with the 1975 Declaration of Helsinki, as revised in 2013.
CONSENT FOR PUBLICATION
The pregnant women were informed about the study and signed the Free Prior Informed Consent Form (FPIC).
STANDARDS OF REPORTING
STROBE guidelines were followed.
AVAILABILITY OF DATA AND MATERIALS
The data which support the findings will be available only after a reasonable request to the corresponding author [R.D.C]. These data are not publicly available due to conditioned restrictions imposed by the ethical committee and pieces of information, which could compromise the participants' privacy.
FUNDING
This study was financed by the Foundation for Research Support of the State of Bahia (FAPESB-Brazil), National Council of Scientific and Technological Development (CNPq – Brazil), and Coordenação de Aperfeiçoamento de Pessoal de Nível Superior – Brasil (CAPES – Finance Code 001).
CONFLICT OF INTEREST
The authors declare they have no conflict of interest.
ACKNOWLEDGEMENTS
Patients and Staff of the Climério de Oliveira Maternity Hospital from the Federal University of Bahia, Brazil.