All published articles of this journal are available on ScienceDirect.
Acute Toxicity and Efficacy of Nanomaterial based Decontamination Formulation Developed for Personal Decontamination against Chemical Warfare Agents
Abstract
Background:
This study addresses the efficacy of nanomaterials based formulation developed for personal decontamination application against chemical warfare agents and used in Personal Decontamination Kit (PDK). It has the potential to decontaminate the skin of an individual, protective equipment, and small arms contaminated with chemical warfare agents. As this formulation has been developed for personal decontamination, risk of nanomaterial toxicity would always be there while sprinkling or applying to the affected area. It may get into the body through various routes specifically through the inhalation route.
Aim:
The aim of this study was to evaluate in vivo decontamination efficiency of the formulation and acute inhalation, intratracheal, intranasal, oral, dermal, and intraperitoneal toxicity of the formulation.
Materials and Methods:
14 days survival was recorded for the evaluation of decontamination efficiency of this formulation. Various endpoints were considered while assessing the toxicity of Nanomaterial Decontamination Formulation which include Organ Body Weight Index (OBWI), serum biochemical parameters, and respiratory variables like tidal volume, respiratory rate, time of inspiration, time of expiration, etc. LD50 of the formulation were also determined for various routes. As skin is the primary organ to come in contact with the decontaminant, its primary skin irritation response has also been determined in this study.
Results and Conclusion:
It was found that there is no gross acute toxicity observed at different doses. Though there were some changes in the initial respiratory pattern, they were all later recovered. The preliminary histological evaluation did not show any adverse effect on various organs after exposure with NDF.
1. INTRODUCTION
Use of chemical warfare agents like sulphur mustard by the military is now an unlikely phenomenon; however, these agents might be used by terrorist or non-state organizations on civilians to create panic and unrest. Presently, no effective antidote is available against sulphur mustard toxicity. Hence, the rapid removal of these agents from exposed skin is the only
valuable way to prevent or decrease tissue damage. Decon-tamination formulation with effective skin decontamination along with detoxification of chemical agent is a challenging task. Various decontaminating formulations have been deve-loped but most of them were not able to detoxify chemical agent. Development of an effective, non-toxic decontaminant for personal decontamination has been the subject of research interest worldwide [1-4].
Fuller’s earth and active carbon have been used for personal decontamination. They are easily available, non-irritating, user-friendly, and cheap so in the past, they were widely acceptable [5]. However, contaminants can be desorbed from them and may contaminate the environment again. On the other hand, chemical decontaminants have been used by India, the Soviet Union, and successor countries, and they lead the world in the development of the new variants and skin-friendly decontamination methods. CC-2, IPP-11 (a mixture of polyethylene glycols and lanthanum nitrate solution), IPP-8 (solution of ethoxyethanol, isopropanol, dimethylformamide, and sulfolane, in which metallic sodium is dissolved before use), potassium ketoxime, and other formulations containing mixture of surfactants with sodium hypochlorite or hydrogen peroxide were in suspension or solution phase [4-6]. Decrease in efficiency with time is a major limitation with suspen-sion/solution decontaminants.
Nanostructured inorganic metal oxides, such as Zinc Oxide (ZnO), Magnesium Oxide (MgO), and titanium dioxide (TiO2), were widely studied and used with good results in chemical warfare agents oxidation and/or degradation reactions [7]. The catalytic performance of inorganic oxides in the decon-tamination is related to their form, size, and shape. When there is any bulk aggregation or contamination by chemical contaminants, these nanomaterial-based formulations are able to remove the hazardous contaminant quite efficiently by physical adsorption and then, they can scarcely degrade and catalytically convert it into nontoxic by-products [8]. Initial studies suggest these decontaminants are found safe to use on skin. In continuation of such studies, we developed a nanomaterial-based decontamination formulation, NDF for personal decontamination. The proposed formulation advan-tageously enables decontamination of an object surface by more than 99% within a minute and simultaneously the formulation causes chemical degradation of about 90% of the adsorbed contaminant within 24 hours (Patent Application has been filed to Indian Patent Office).
As this formulation is developed for personal decon-tamination, risk of nanomaterial occupational or accidental toxicity is always there. It would be obvious to inhale aerosol generated by the decontamination formulation or to come in contact with skin while sprinkling from pressure bottles to the affected area. It may possibly generate dust which may create respiratory effects or skin irritancy. Inhalation may not be the only route to get accidental exposure; it can enter our body through any route like oral, dermal, or any parental route. The aim of this study is to evaluate its acute dust inhalation toxicity, skin irritancy response, and acute oral, dermal, and intraperitoneal toxicity. In this study, bio-efficacy and acute toxicity of nanoparticle-based formulation NDF has been evaluated which was developed for personal chemical agent decontamination.
2. MATERIALS AND METHODS
2.1. Chemicals
Magnesium acetate tetrahydrate, zinc acetate dihydrate, oxalic acid dihydrate, acetonitrile, dichloromethane (DCM), titanium tetrachloride, isopropanol, and ethanol were obtained from E. Merck India Ltd. Sulphur Mustard {SM; bis-(2-chloroethyl) ethyl sulphide} was synthesised in the Synthetic Chemistry Division and was found to be above 99% pure by gas chromatographic analysis. General chemicals were purchased from Sigma (USA) with analytical grade. Biochemical kits were from E-coline Merck Pvt ltd. (India).
2.2. Animals
Randomly bred Swiss female mice (25 - 30 g) from the Institute's animal house facility were used for the evaluation of decontamination efficiency of NDF. Acute toxicity studies were done in randomly bred male Wistar rats. Primary skin irritation test was done on a New-Zealand rabbit. All the animals were kept in polypropylene cages with sterilised and dry paddy husk as a bedding material. Free access to food (Ashirwad Ltd, India) and water allowed until two hours before the experiment. The care and maintenance of the animals were as per the approved guidelines of the 'Committee for the Purpose of Control and Supervision of Experiments on Animals (CPCSEA)', India. A day before percutaneous exposure, hair on the back of the animals was closely clipped using a pair of scissors. Food and water were allowed two hours after the experiment. All animal procedures were approved by the Institutional Animal Ethical Committee.
2.3. Synthesis and Characterization of NDF
NDF was prepared by thoroughly mixing 90% TiO2 nanoparticles of 5-15 nm size range, 8% MgO nanoparticles of 5-15 nm size range, and 2% ZnO nanoparticles of 20-30 nm size range with the help of planetary ball mill. SEM data of NDF was recorded on Quanta 400 ESEM of FEI, The Netherlands make. TEM measurements were done using a field emission transmission electron microscope Tecnai TEM, FEI, The Netherlands. The sample was suspended in acetone and sonicated for 10 min prior to the fabrication of grids for TEM analysis. XRD patterns were obtained by X Pert Pro Diffractometer, Panalytical, The Netherlands make using Cu Kα radiation. Nitrogen adsorption measurements were done on ASAP 2020 surface area analyzer of Micromeritics, the USA make.
2.4. In vivo Decontamination Efficiency of NDF
Female mice were clipped one day before to start the experiment. On the day of the experiment, they were randomly divided into 3 groups containing four mice in each: group 1 (percutaneously applied SM but not decontaminated), group 2 (percutaneously applied SM followed by NDF decon-tamination), and group 3 (percutaneously applied SM followed by Fuller’s earth decontamination). Survival and body weight change were recorded for 14 days in all the groups.
2.5. Primary Skin Irritation Test of NDF
A New-Zealand white rabbit was used for this study. Hair from flanks (4”×6” dorso-caudal area) of the rabbit was closely clipped by a pair of scissors and sponged with moist cotton in 0.9% NaCl one day before the experiment. Four areas (each area = 1 inch2 approximately) of skin, two on right side of the rabbit’s back were utilised for application of NDF (0.5 grams at each site), two on left were kept as control, exposed with talcum powder (0.5 grams at each site). Out of four sites, two were abraded with criss-cross scratching made by a sharp needle, one for control and the other for test. NDF was applied on aforesaid skin sites. Thereafter, the skin sites were covered with PARAFILM “M” and gauze which was place by adhesive tape (Johnson and Johnson) and the animals were immobilized by using rabbit restrainer for 4 hours. For comparison, the adjacent left sites of the skin were exposed to talcum powder as control covered similarly. After 4 hours, PARAFILM “M”, gauze, and adhesive tape were removed, the sites were cleaned using moist cotton swabs in 0.9% NaCl and allowed to dry for 5 minutes. Thereafter, each of the skin sites was carefully inspected and scored as per the standard procedure for erythema and edema.
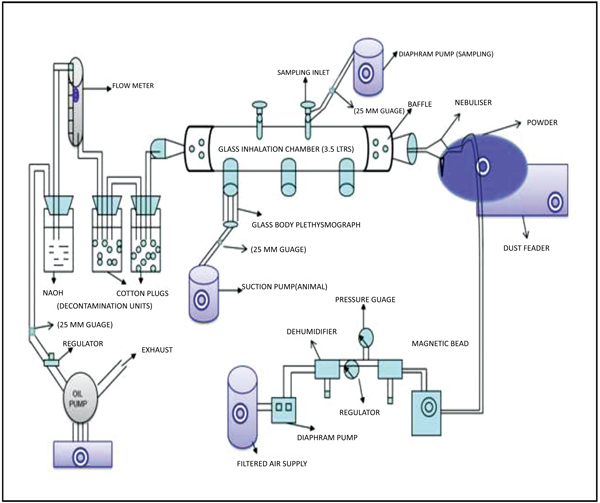
2.6. Dust Inhalation Toxicity of NDF
Dust Inhalation toxicity of NDF was carried out in male Wistar rats. Four animals in each group were randomly allocated to five different groups as follows. Group I: Fuller’s earth (5 gm/hr); Group II: NDF (5 gm/hr); Group III: NDF (10 gm/hr); Group IV: NDF (20 gm/hr); Group V: NDF (40 gm/hr).
A head-only inhalation exposure chamber made of glass (3.5 litres volume) attached with glass nebulizer connected with dust feeder was fabricated at DRDE, Gwalior. NDF dust was nebulised under fixed conditions at 2.5 psi (pounds per square inch at the inlet of the nebuliser) with the chamber airflow rate of 20 LPM (litres per minute). Temperature and humidity were maintained in the chamber. The outgoing air passed through traps with cotton balls followed by sodium hydroxide solution for the removal of NDF before exit in the environment. Rats were restrained in glass body plethys-mographs connected with volumetric flow pressure transducers (Grass, PT-5, USA). The transducers were connected with the amplifier (Universal Amplifier Gould, USA) and finally, the digital response was observed in an oscilloscope (WindoGraf 930, USA). The small air pressure changes recorded by the transducers were then amplified and then these amplified signals were sent to an oscilloscope and computer through an analogue digital converter card (KeithleyMetrabyte, Model DAS-16, USA). The oscilloscope recorded these signals intermittently and displayed the respiratory pattern of the rats in sigmoid shape whereas the computer with the help of a program specifically for this purpose computed the respiratory variables (Fig. 1). This program records signals every 15 seconds interval and the duration of 15 seconds is called ‘data period’. Sigma Plot 2.0 (SPSS, Chicago, USA) was used for plotting the graphs [9]. Various respiratory variables such as respiratory Tidal Volume (VT), Frequency (F), Dead Volume (VD), and Time of Inspiration (TI), Time of Expiration (TE), Time of Break (TB), and Time of Pause (TP) were recorded at various time points.
All the rats were first acclimatized in body plethysmograph for 30 minutes. After acclimatization, initial respiratory data (pre-exposure control) were collected without dust exposure for 30 minutes. Same animals were then exposed with NDF dust for 4 hours followed by 30 minutes of recovery. All the rats were kept under observation for 14 days, survival, body weight change, and gross behavioral changes were recorded.
2.7. Acute Toxicity of NDF through Various Routes
Acute toxicity of NDF was done through various routes including oral, dermal, intra-peritoneal, intra-tracheal, and intra-nasal in male Wistar rats.
2.7.1. Acute Oral and Dermal Toxicity of NDF
Male Wistar rats were fasted for four hours before oral administration. Ten rats were randomly divided into two groups (five in each group): Group1 - control group (administered 0.1% Hydroxyl Propyl Cellulose (HPC) and Group2 - exposed group (administered NDF suspension in 0.1% HPC after sonication at the dose 5 g/kg body weight). For dermal toxicity, male Wistar rats were clipped on the day before the experiment and divided into two groups as mentioned above. Animals were exposed to 2g/kg body weight dose with the help of cotton gauze and adhesive tape. After 24 hours the gauze and adhesive tape were removed and the animals were observed for erythema and edema and scored as per the standard procedure. The symptoms, body weight, and mortality were observed and recorded for 14 days. On the 14th day, all animals were first weighed and then sacrificed. Liver, lungs, spleen, heart, and kidneys were excised and weighed for OBWI. Blood was collected by the retro-orbital route and biochemical estimations like glucose, cholesterol, triglycerides, and total protein were done for toxicity assessment.
2.7.2. Acute Toxicity of NDF Administered through Intra-peritoneal Route
Eight Swiss albino male mice were randomly divided into two groups (four in each group): Group1 - control group (administered phosphate buffer saline pH 7.4) and Group2 - exposed group (NDF in phosphate buffer saline (pH 7.4) at the dose of 570 mg/kg body weight [highest dose achieved for intra-peritoneal route]. The symptoms, body weight, and mortality were observed and recorded for 14 days. On the 14th day, all animals were weighed and sacrificed after being anesthetized by anesthetic ether. Blood was collected by the retro-orbital route. Liver, lungs, spleen, heart, and kidneys were excised and weighed for OBWI. Liver and spleen were kept for histological examination.
2.7.3. Acute Toxicity of NDF Administered through Intra-tracheal and Intra-nasal Route
For intratracheal administration, 8 male Wistar rats were randomly divided into two groups (four in each): Group1 - control group (administered phosphate buffer saline (pH 7.4), 200µl) and Group2 exposed group (administered 200 µl of NDF suspension in phosphate buffer saline (pH 7.4) after sonication at the dose of 100 mg/kg body weight). For intranasal administration, 8 Swiss male mice were randomly divided into two groups (four in each): Group1 - control group (administered phosphate buffer saline (pH 7.4), 10µl in each nostril) and Group2 exposed group (NDF suspension administered at the dose of 100 mg/kg in 10µl in each nostril). Animals were then sacrificed and samples were collected for further evaluation.
2.7.4. Histology Protocol
After sacrificing the animals, various organs were taken out and fixed with bouin’s fluid for fifteen days. Tissues were processed as per standard histology protocol. Finally, thin sections of 5µm were cut and placed on the slides. All the slides were mounted and stained with H & E staining and examined [10].
2.8. Statistical Analysis
Results were expressed as mean ± Standard Error (SE). For statistical analysis, each experimental value was compared with its corresponding control using student’s t-test. In the inhalation groups, mean values at different time points were compared with control mean values by one-way Analysis of Variance (ANOVA) test. Statistical significance for all tests was set at P<0.05.
3. RESULTS
3.1. Synthesis and Characterization of NDF
The TEM image of NDF is shown in Fig. (2). It shows nanoparticles of NDF were of spherical shape and size ranging from 5 to 30 nm to a major extent. These nanoparticles are found to have been aggregated due to van der waals attractive forces. SEM image (Fig. 3) also illustrates the aggregates of spherically shaped nanoparticles of size less than 100 nm. The XRD pattern of NDF is shown in Fig. (4). XRD pattern shows peaks at 25.36 (100), 37.92 (004), 47.82 (200), 54.62 (106), and 62.88 (215) and indicates the presence of TiO2 nanoparticles of anatase phase. It also depicts the peaks at 31.65 (100), 34.25 (002), and 36.09 (101) indicating the presence of ZnO nanoparticles of zincite phase and shows peaks at 42.867(200) and 62.184(220) indicating the presence of MgO nanoparticles of periclase phase. The crystallite sizes of TiO2, MgO, and ZnO were found to be 5.6 nm, 11 nm, and 30 nm, respectively, as per Scherrer analysis of the data and the same is consistent with TEM observations.
3.2. Decontamination Efficiency of NDF against SM Exposure
All the control animals not decontaminated with NDF died within 24 hours at 20 µl dose of neat sulphur mustard but after decontamination with NDF, the animals’ survival time was increased. At 20 µl of neat sulphur mustard, two animals died out of four in the NDF decontaminated group and in case of fuller’s earth, all four animals died. This shows that the nanoparticles based formulation has better efficiency than the fuller’s earth to decontaminate sulphur mustard (Table 1).
Decontaminants |
SM Neat (5 µl) |
SM Neat (10 µl) |
SM Neat (20 µl) |
---|---|---|---|
SM control (no decontamination) |
4/4* | 4/4 | 4/4 |
SM + NDF (nanoparticles) |
1/4 | 2/4 | 2/4 |
SM + Fullers Earth (FE) |
0/4 | 2/4 | 4/4 |
3.3. Acute Toxicity of NDF through Various Routes (Oral and Dermal)
Survival of male Wistar rats was observed for 14 days after oral and dermal administration, no mortality and other signs of toxicity were observed in these routes. It was found that after oral ingestion of NDF, animals become dysenteric initially for 1 to 4 days but later recovered. After dermal exposure of NDF at 2 g/kg dose, no significant erythema and edema were observed. LD50 was found to be more than 5 g/kg for oral and 2 g/kg for dermal routes. The primary skin irritancy test showed that the nanoparticles based formulation was a negligible irritant to the rabbit skin at both the sides abraded and non-abraded as there was no erythema and edema observed in the non-irritant range, PSII for the formulation is less than 0.5 (Table 2).
Route | LD50 |
---|---|
Oral | >5 g/kg |
Dermal | >5 g/kg |
Intraperitoneal | >570 mg/kg |
Intratracheal | >100 mg/kg |
Intranasal | >100 mg/kg |
Primary skin irritation index (PSII) |
0.5 |
3.4. Changes in Body Weight, OBWI and Biochemical variables after Exposure/Administration of NDF
Various biochemical parameters have been done on the plasma samples collected from the control and exposed animals through oral and dermal routes. After oral and dermal exposure with NDF at a dose of 5 g/kg, there was no significant difference (P<0.05) between control and exposed group. The values of biochemical parameters were within range and not significantly different from the control values. This study showed that there is a significant decrease (P<0.05) in the OBWI of heart after 14 days of oral exposure at 5 g/kg of NDF in Wistar rats when compared to control rats. In inhalation exposure of NDF, there is a significant decrease (P<0.05) in the OBWI of kidney when compared with its respective control group. Remaining groups showed no significant difference (P>0.05) in any other OBWI (Tables 3 and 4).
Routes | Groups | Doses | Organs | ||||||
---|---|---|---|---|---|---|---|---|---|
Liver | Lungs | Spleen | Heart | Kidneys | |||||
Dermal (14 days) |
Control | - | 3.37±0.31 | 0.71±0.02 | 0.3±0.06 | 0.38±0.01 | 0.69±0.04 | ||
Treated | 5 g/kg | 3.32±0.03 | 0.69±0.05 | 0.34±0.01 | 0.3±0.02 | 0.62±0.03 | |||
P value | 0.878 | 0.723 | 0.535 | 0.3±0.02 | 0.211 | ||||
Intratracheal | Control | - | 3.56±0.15 | 1.00±0.01 | 0.47±0.04 | 0.31±0.00 | 0.62±0.03 | ||
Treated | 100mg/kg | 3.29±0.22 | 1.10±0.12 | 0.26±0.02 | 0.3±0.01 | 0.6±0.03 | |||
P value | 0.368 | 0.453 | 0.009* | 0.374 | 0.662 | ||||
Intranasal | Control | - | 4.89±0.27 | 1.29±0.04 | 0.68±0.09 | 0.44±0.02 | 1.01±0.02 | ||
Treated | 100mg/kg | 5.05±0.37 | 1.53±0.12 | 0.48±0.05 | 0.48±0.05 | 1.09±0.06 | |||
P value | 0.739 | 0.107 | 0.100 | 0.486 | 0.253 | ||||
Intraperitoneal | Control | - | 5.58±0.17 | 1.07±0.02 | 0.65±0.06 | 0.43±0.03 | 0.93±0.04 | ||
Treated | 570mg/kg | 6.26±0.25 | 0.95±0.12 | 0.52±0.04 | 0.49±0.01 | 1.05±0.03 | |||
P value | 0.066 | 0.362 | 0.121 | 0.107 | 0.053 | ||||
Oral | Control | - | 2.963 ±0.205 |
0.758 ±0.031 |
0.382 ±0.038 |
0.414 ±0.033 |
0.625 ±0.036 |
||
Treated | 500g/kg | 3.174 ±0.039 |
0.765 ±0.055 |
0.328 ±0.033 |
0.311 ±0.006 |
0.581 ±0.018 |
|||
P value | 0.232 | 0.928 | 0.345 | 0.007* | 0.266 | ||||
Inhalation | NF | 40 g | 3.103 ±0.153 |
0.825 ±0.018 |
0.404 ±0.052 |
0.372 ±0.005 |
0.588 ±0.032 |
||
P value | 0.965 | 0.267 | 0.698 | 0.029* | 0.0533 |
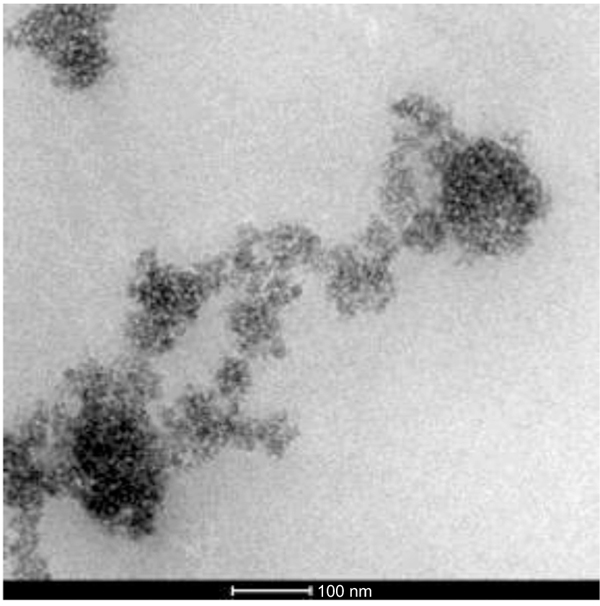
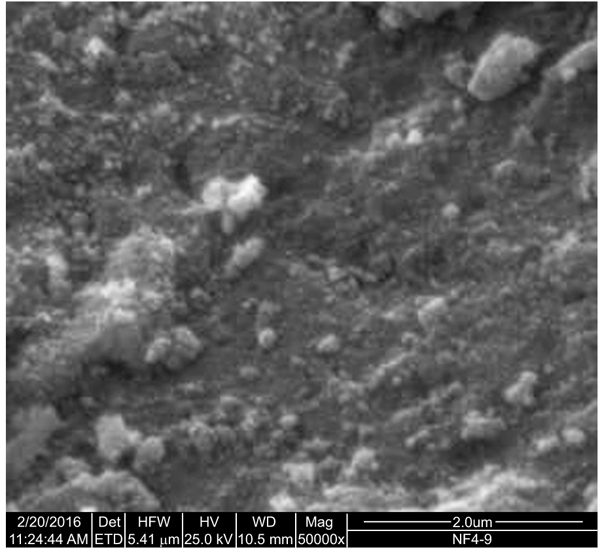
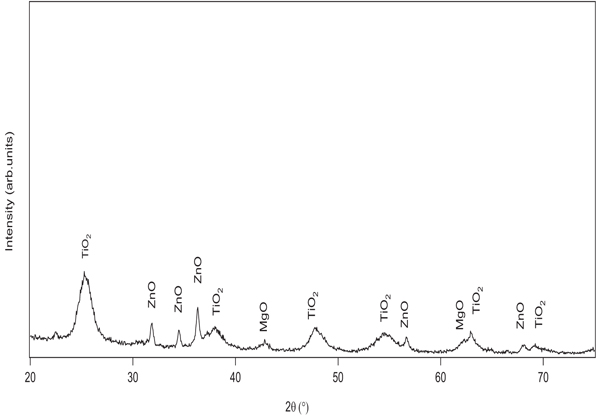
Biochemical Parameters |
Oral (5 g/kg) |
Dermal (2 g/kg) |
Intra-peritonial (570 mg/kg) |
Intratracheal (100 mg/kg) |
Intranasal (100 mg/kg) |
---|---|---|---|---|---|
%AST | 74.4±7.5 | 96.4±6.3 | 137.8±57.4 | 193.7±40 | 180.6±47.1 |
%ALT | 69.9±7.5 | 95.2±5.2 | 100.9±23.4 | 94.9±6.1 | 157.8±12.7 |
%ALP | 100 ± 12.3 | 99.9±24.5 | 99.9±27.2 | 161.1±17.3 | 49.7±8.3 |
%LDH | 161.7±78.2 | 119.1±119.1 | 113.6±13.6 | 332.8±100 | 139.9±12.0 |
%BUN | 118.3±16.9 | 93.1±6.1 | 131.5±44.4 | 115.6±17.6 | 100.7±21.7 |
%CR | - | 93.5±9.7 | 95±5 | 100±0 | 58.3±11.0 |
%Glucose | 98.6±9.7 | 119.6±8.3 | 113.2±11.9 | 128.8±17.9 | 90.6±2.03 |
%Protein | 114.8±7.0 | 105.4±2.0 | 98.4±5.9 | 99.8±0.7 | 101.4±7.1 |
%Triglycerides | 98.8±27.4 | 111.9±24.8 | 170.8±87.0 | 118.4±118.4 | 117.3±15.5 |
%Cholesterol | 115.9±17.2 | 88.7±6.8 | 104.1±106.5 | 114.6±15.5 | 85.5±4.2 |
3.5. Changes in Respiratory Variables after Acute Dust Inhalation, Acute Intratracheal and Acute Intranasal Exposure/Administration of NDF
Various respiratory variables were recorded at different time points after intranasal administration of NDF suspension in phosphate buffer saline (pH 7.4), and compared it with the control animals. These results showed that though there is an increase in tidal volume, time of inspiration, time of expiration, and decrease in frequency, the change was non-significant and all the values tend to normalize once exposure stopped in 4 hrs (Fig. 5). Time of break and time of pause was significantly increased at initial time points after intranasal administration of NDF but these values also normalized within 6 hrs of exposure. Same results were found with intratracheal administration. Various respiratory variables were recorded at different time points after intratracheal administration of NDF suspension in phosphate buffer saline (pH 7.4) and compared it with the healthy control group. The change in various respiratory variables was not significant (P >0.05) and recovered once the exposure terminated (Fig. 6).
In inhalation exposures, animals were exposed to different concentrations of dust. There was no mortality in any test concentration while fuller’s earth exposed animal at 1.65 g/m3/min showed one death out of four animals in 14 days observation (Table 5). At maintained temperature 23 ± 0.09 oC and humidity 45.0 ±0.6%, inhalation exposure of NDF dust showed decrease in tidal volume, time of inspiration, time of expiration, and increase in frequency as concentration increases. Time of break, time of pause, and dead volume were increased at initial time points after inhalation exposure of NDF but these values also normalized in 14 days (data not shown). Significant changes were noted in tidal volume and frequency in NDF at 3g/m3/min concentration (P-value = 0.042*) and in time of inspiration and expiration (P-value = 0.001* and 0.009*), respectively (Fig. 7). Changes in respiratory variables normalized in 14 days (Fig. 8). The respiratory pattern also showed sensory irritation in the upper respiratory tract which also tends to normal once the exposure terminated (Fig. 9).
3.6. Gross Changes in the Histology of Different Organs after NDF Exposure/Administration
Histology of various organs was done after NDF administration through different routes. There were no alterations in skin histology after dermal exposure at 5 g/ kg dose. Cell structure was intact and no inflammatory cells were seen in exposed skin. After intratracheal and intranasal exposure, lung histology was intact and fibrosis and inflam-mation were not observed in any group. Through the intra-peritoneal route, NDF was little detrimental as some apoptotic and necrotic cells were seen in liver as well as in spleen when compared to control group but remaining histology is normal. After oral ingestion of NDF mucus secretions were increased in stomach on the 14th day of oral feeding. Intestine histology was found normal and intact in exposed group after oral ingestion of NDF (Fig. 10).
Group |
Nominal Concentrations (g/m3/min) |
Amount of Dust Inhaled (mg/rat)$ |
Animal Died# |
---|---|---|---|
Control (FE) | 1.65 | 63 | 1/4^ |
NDF-5 g | 0.95 | 36 | 0/4 |
NDF-10 g | 1.90 | 73 | 0/4 |
NDF-20 g | 3.00 | 115 | 0/4 |
NDF-40 g | 4.55 | 175 | 0/4 |
4. DISCUSSION
Efficacy and acute toxicity of NDF were investigated in this study through different routes viz oral, dermal, intaperi-toneal, intratracheal, intranasal, and inhalation. NDF is a combination of three different types of nanoparticles with different characteristics namely titanium dioxide, zinc oxide, and magnesium oxide at different percentages. This decon-tamination formulation was prepared by mixing 90% of TiO2 nanoparticles of 5-15 nm size range, 8% of MgO nanoparticles of 5-15 nm size range, and 2% of ZnO nanoparticles of 20-30 nm size range. Prepared formulation exhibited very high phy-sical removal efficiency on contaminated surfaces. Decon-tamination studies on mice confirmed its efficiency to remove chemical warfare agents from contaminated skin. NDF can also chemically degrade sulphur mustard and sarin unlike Fuller’s earth (data not shown). Fuller’s Earth (FE) already had been widely accepted as decontaminating clay and reported with high surface area and large capacity to absorb toxic chemicals. It has been used in Personal Decontamination Kits (PDK) against CWA contaminated surfaces. Fuller’s earth has also been used for the decontamination of individual protective equipment and small arms at first aid stations, field conditions, and laboratory sites where CWA contamination takes place. But the major drawback with fuller’s earth was the disposal of contaminated fuller’s earth which may release the chemical agent in the environment after some time. To overcome this limitation, a formulation has been designed which can neutralize chemical warfare agent up to great extent so that the risk of re-exposure could be minimized. As this formulation has been developed for the personal decontamination of chemical warfare agents, it is expected to have toxicity of these nanoparticles which they individually exhibit.
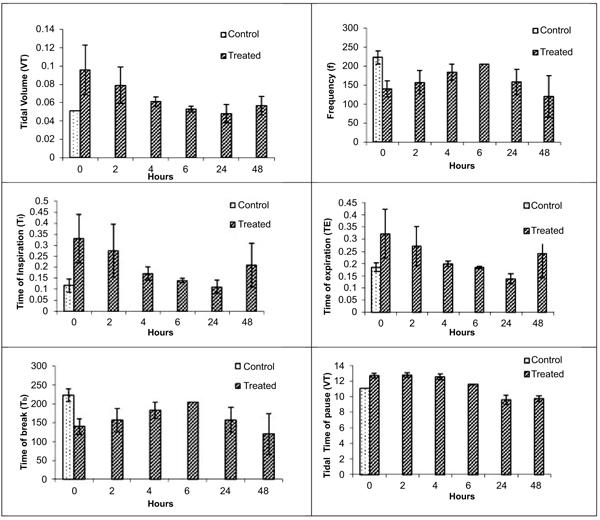
VT (Tidal volume), f (Respiratory frequency), TI (Time of inspiration), TE (Time of expiration), TB (Time of break), TP (Time of pause). Values represent means ± SE, n=4. P < 0.05 (One way ANOVA), as compared to healthy control group.* Values significantly differ from the healthy control value at 0 hour.
Various earlier reports suggested the TiO2 may exhibit toxicity through various routes. Intragastric administration of NDF at limit dose resulted in dysentery at initial days from day 1 to day 4 but at later days it was recovered. Histology of stomach and intestine showed mucous secretion in the alimentary canal at the 14th day of post oral exposure. It may be due to the activation of free radical generation and a decrease in the stress-related gene expression levels of superoxide dismutase, catalase, glutathione peroxidase, metallothionein, etc [11] in vitro ROS production, ER activation, and pro inflammatory mediator production (TNFα and MIP-2) were also reported for TiO2 [12]. Histopathology of liver and spleen after intrapertonial injection of nanoparticle also showed increased apoptotic cells and congestion. Mild degenerative changes with deposition of brown pigment hemosiderin were observed in spleen sections was also indicative of free radical-induced degeneration. Formulation directly absorbed in various organs created maximum degenerative changes in histology of day 14 organ samples. The maximum administrable dose of this formulation through the intraperitoneal cavity was 570 mg/kg; above this clumps were formed and animals also showed writhing movement. It was reported that after intraperitoneal injection of TiO2 showed depression, anorexia, diarrhoea, lethargy, tremor, body-weight loss, can cause lesions in organs i.e. spleen, liver, lungs, and kidneys but spleen had the highest lesions [13]. It is assumed that detrimental effect of this formulation through the parental route may be due to the presence of TiO2 as one of the major component. An oxidant driven response was integral to the toxicity of TiO2, which had inflammatory and cytotoxic consequences [14]. ZnO nano-particles were also reported for edema and degeneration of hepatocytes, and inflammation of pancreas in mice treated with 20 nm ZnO through the intraperitoneal route. Oral feeding of NDF does not cause any toxicity. It was observed that after 14 days of oral dosing, GOT and GPT levels went down but within range, and also it does not have any clinical mani-festation. Through the dermal route, NDF is safe and there is no toxicity finding via dermal route
Intratracheal instillation of nano-sized zinc oxide (N-ZnO) in mice showed pulmonary inflammation, proliferation, and thickening of alveolar walls. ZnO takes a small fraction of this formulation, so its effect on the lungs was not visible in this study. ZnO nanoparticles were also found to induce oxidative stress in cells indicated by depletion of glutathione (59% and 51%); catalase (64% and 55%) and superoxide dismutase (72% and 75%) at 0.8 and 0.08 μg/ml respectively [15]. It is expected that ZnO might also contribute to the histological changes appearing after intraperitoneal injection [16, 17]. Zinc oxide or Titanium oxide nanoparticles do not penetrate the stratum corneum so the chance of dermal toxicity by formulation is very rare [18]. Possibility of these nanoparticles to reach deeper epidermal layer through transfollicular of transglandular routes only [19]. Toxicity studies of magnesium oxide nanoparticles were not done so elaborately but it may be expected that these nanoparticles will show bowel obstruction after long term use of magnesium oxide [20].
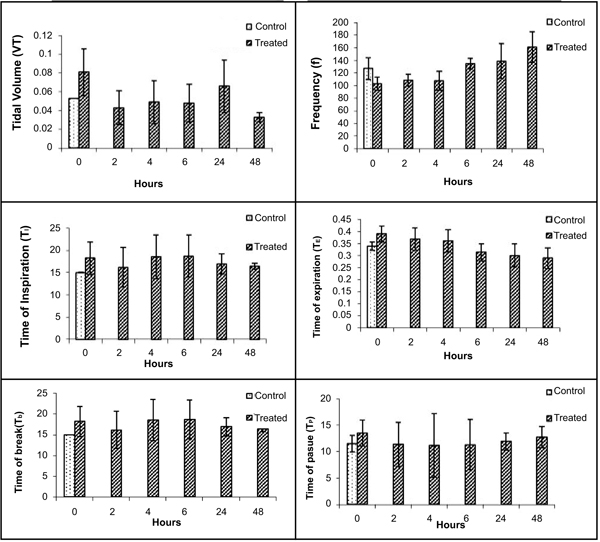
VT (Tidal volume), f (Respiratory frequency), TI (Time of inspiration), TE (Time of expiration), TB (Time of break), TP (Time of pause). Values represent means ± SE, n=4. P < 0.05 (One way ANOVA), as compared to healthy control group.* Values significantly differ from the healthy control value at 0 hour.
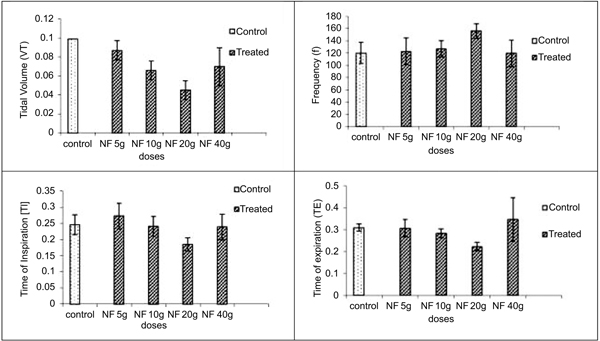
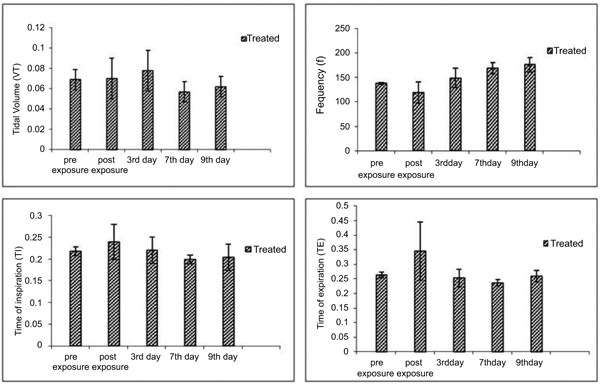
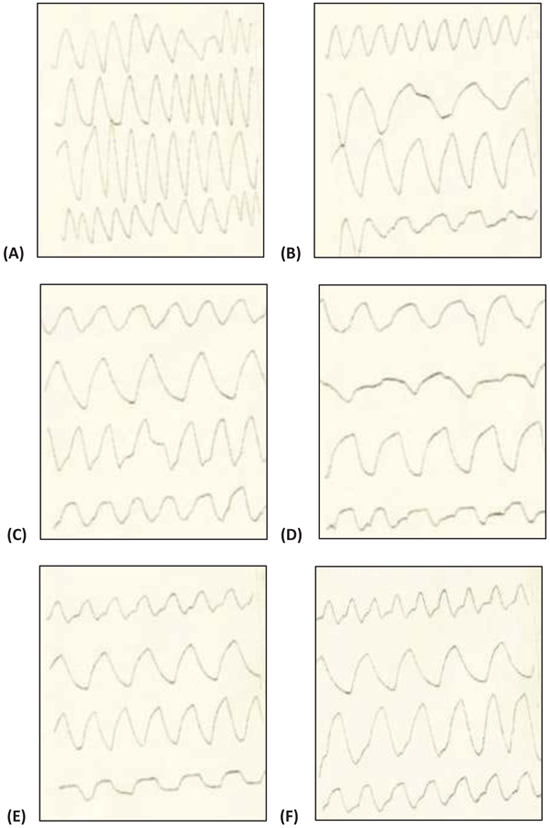
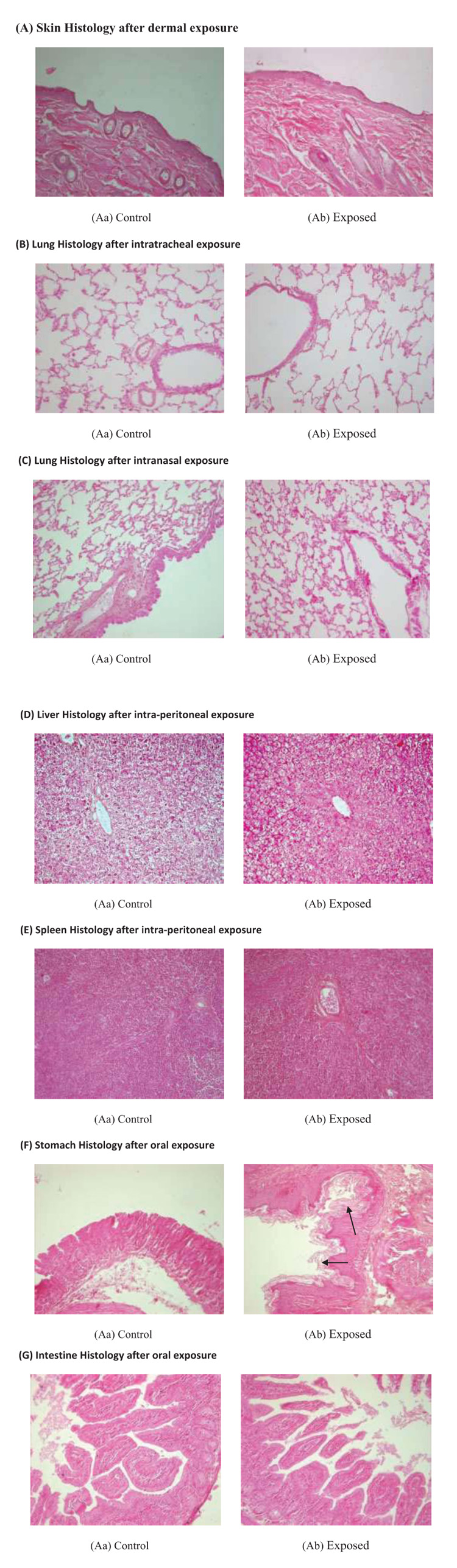
There are various alternative routes available for the delivery of NDF to the lungs which include intratracheal administration, Intranasal (IN) aspiration, whole-body inhalation or nose-only inhalation in a specially designed apparatus, each of this delivery mode has their own pros and cons. Rodents are nose breathers so delivery to the entire administered dose to the deep lungs is only possible through intratracheal administration [21]. Intranasal aspiration is simpler to perform and felt to be a more toxicologically relevant route for particle administration. Particles follow the same path as followed in the inhalation route but actual dose may be different than the expected dose due to impact on nasal wall and administration of single dose. It is reported that only about 33% of the particle dose presented to mice for intranasal aspiration actually gets to the lung. The remainder is either removed during passage through the nasopharyngeal region or by sneezing and other maneuvers by the animal to clear the nose of foreign substances being introduced [22]. Nose-only exposure requires less material than whole-body exposure, introduces increased animal handling which may affect the experimental outcome but it helps to understand the effect of particle under 3-4 hours continuous exposure which is not possible with intranasal aspiration. In view of this, it is required to generate data of respiratory variables by using all three alternatives to understand the changes in respiration after exposure of NDF.
Reduction in spleen organ body weight index after intratracheal exposure of NDF may be due to the increased phagocytic activity during the transfer of nanoparticles and these phagocytes may relocate in spleen and cause apoptosis or necrosis in spleen [23]. The intratracheal route also showed increase in LDH values along with AST which indicates the possibility of mild myocardial infarction. There was no significant difference in any of the respiratory variables shown after intratracheal administration because of no adherence or accumulation of nanoparticles in lungs. It also does not cause any irritation or airway obstruction. Intratracheal adminis-tration of NDF showed mild biochemical alterations and degenerative changes due to more absorption of nanoparticles to the system as administered directly in the lungs bypassing upper respiratory tract. After intranasal exposure of NDF, these observations (increase in LDH levels and decrease in cholesterol levels) suggest that there is a possibility of pernicious anemia. It is reported that only about 33% of the particle dose presented to mice for intranasal aspiration actually gets to the lung. The remainder is either removed during passage through the nasopharyngeal region or by sneezing and other maneuvers by the animal to clear the nose of foreign substances being introduced. Respiratory variables changed in initial phase of exposure up to 3-4 hrs but normalized in 6 hrs. Changes in these parameters showed there may be some sensory irritation initially but not prolonged. 4 hrs dynamic exposure of NDF dust showed sensory irritation. Remaining parameters were within range and do not show any change from the control group. These initial experiments showed that NDF is safe and can be used further. In case of accidental exposure of large quantities of these nanoparticles, individual has to be immediately removed from exposure. It will be helpful to regain normal lung physiology.
CONCLUSION
It may predict that the alterations which we have observed may be due to oxidative stress and apoptosis caused by component nanoparticles. These results suggest that though there are few possibilities of certain clinical manifestations after inhalation of nanoparticles, its toxicity levels are very low and it is safe up to 5 g/kg and 2 g/kg dose through oral and dermal route respectively. These results suggest that NDF formulation can be taken further for decontamination formu-lation development. Further studies of sub-acute toxicity and sub-chronic toxicity may be required for better clarification of expected toxicity and clinical manifestation.
ETHICS APPROVAL AND CONSENT TO PARTICIPATE
All procedures have been approved from DRDE Institutional Animal Ethical Committee.
HUMAN AND ANIMAL RIGHTS
No humans were used for studies that are the basis of this research. The care and maintenance ofthe animals were as per the approved guidelines of the' Committee for the Purpose of Control and Supervision of Experiments on Animals (CPCSEA)'.
CONSENT FOR PUBLICATION
Not applicable.
AVAILABILITY OF DATA AND MATERIALS
Not applicable.
FUNDING
None.
CONFLICT OF INTERESTS
The authors declare no conflict of interest, financial or otherwise.
ACKNOWLEDGEMENTS
Authors are greatful to the Director DRDE, gwalior for motivating to carry out these studies.