All published articles of this journal are available on ScienceDirect.
A Recent Update on Candidate Biomarkers in the Pathogenesis of Diabetic Retinopathy
Abstract
Background
Diabetic Retinopathy (DR), a retinal vascular disease caused by hyperglycemia, is the most common microvascular consequence of diabetes that affects a significant proportion of young adults. The transition from the non-proliferative to the proliferative stage results in vitreous hemorrhage, retinal detachment, and ultimately irreversible blindness. Currently, there are no effective interventions to support the early treatment of diabetic retinopathy. Non-invasive methods, such as AI-mediated deep learning, are useful for screening and diagnosing DR once visual changes become evident. These methods also help assess whether current treatments are improving vision. Furthermore, existing pharmaceutical therapies are typically employed only when vision is already impaired. Therefore, to predict the onset of the disease, monitor its progression, and identify new therapeutic targets, it is crucial to search for optimal biomarkers that have high specificity and sensitivity related to the pathogenesis of DR.
Aim
This narrative review aims to address the regulation of circulating biomarkers in DR, with a particular emphasis on its pathogenesis, which includes inflammation, oxidative stress, angiogenesis, and neurodegeneration, as well as the associated therapies. It also discusses the role of nutritional factors in DR and highlights studies conducted on human populations to date.
Methodology
A review was conducted using databases from the Cochrane Library and search engines such as PubMed, Google Scholar, Research Gate, and Scopus. The study included Type 2 Diabetes Mellitus (T2DM) with or without diabetic retinopathy. Exclusion criteria includedstudies conducted in Gestational diabetes mellitus, Type 1 Diabetes mellitus, editorial, pilot studies, conferences, abstracts, interviews, thesis, and unpublished work.
Results: Based on the putative molecular targets of these biomarkers, future research may successfully create novel therapeutics to reduce the overall burden of the disease and enhance the visual outcome of diabetic patients
Conclusion
Ultimately, this could improve patients' quality of life by reducing the impact of vision loss and alleviating the overall burden of the disease.
1. INTRODUCTION
The estimated global prevalence of type 2 diabetes mellitus in 2019 among individuals aged 20–79 years was 4.63 million, or 9.3% of the population. By 2045, the estimated number of cases is expected to rise to 10.9%, or 700.2 million [1]. The increasing prevalence of diabetes can lead to a rising rate of associated vascular complications such as DR. This retinal vascular disease caused by hyperglycemia is the leading cause of irreversible vision loss among working adults [2]. A cross-sectional survey based on data from the SMART India Study 2022 found that the estimated national prevalence of blindness was 2.4% (95% CI: 1.7-3.4). Additionally, the prevalence of vision impairment was reported at 21.1% (95% CI: 15.7-27.7). Among individuals diagnosed with diabetes, blindness was significantly associated with diabetic retinopathy (aOR: 3.06, 95% CI: 1.25-7.51), vision-threatening diabetic retinopathy (aOR: 7.21, 95% CI: 3.52-14.75), and diabetic macular edema (aOR: 5.41, 95% CI: 2.20-13.33) [3]. There is a significant financial burden associated with vision impairment. Among the 2.2 billion people worldwide, 94 million have cataracts, 88.4 million have refractive errors, 8 million have age-related macular degeneration, 7.7 million have glaucoma, and 3.9 million have DR [4].
The modifiable risk factors associated with the development and pathophysiology of the disease are hypertension, dyslipidemia, and hyperglycemia. Other manageable risk factors are age, inadequate glycemic control, and the duration of diabetes. To identify the fundamental cause of an illness, it is critical to comprehend its etiology. When retinal capillaries show signs of edema, hemorrhage, or microaneurysms, it is classified as Non-Proliferative Diabetic Retinopathy (NPDR). When fibrous connective tissue grows abnormally on the retina, it is classified as Proliferative Diabetic Retinopathy (PDR), and this stage of visual impairment is irreversible [5]. One of the primary concerns is that patients remain asymptomatic until visible symptoms appear, so active treatment is initiated only at the advanced stages of the disease. Moreover, patients with diabetic retinopathy are also at higher risk for developing cardiovascular disease and diabetic nephropathy, among other vascular complications [6, 7] The progression of ocular damage and vascular complications can be prevented once the disease is identified as early as possible.
Techniques such as AI-mediated deep learning help diagnose and assess the risk of retinopathy screening. Despite being non-invasive, these methods are limited to detecting underlying visual changes and evaluating the potential impact of interventions on visual function. They only target the eye once it has already been affected by the disease. Therefore, there is a need for an ideal biomarker that is highly sensitive and focused on the pathogenesis of DR. Such a biomarker would be valuable for predicting the disease, tracking its progression, and identifying new therapeutic targets. In this context, we aimed to examine the reported circulating biomarkers that are related to DR, with a specific emphasis on their role in the disease's pathogenesis. Additionally, we explored the involvement of nutritional factors in DR and highlighted studies conducted on human populations.
2. METHODOLOGY
2.1. Database Search Strategy
The literature was evaluated using search engines such as PubMed, Google Scholar, Scopus, and the Cochrane Library databases. We selected articles for analysis based on the presence of key terms such as diabetes mellitus, oxidative stress, inflammation, neurogenesis, angiogenesis, biomarkers, diabetic retinopathy, and combinations of these terms. The most recent research, outstanding papers, meta-analyses, and English-language articles were prioritized in the literature. The majority of included studies (90%) were published after 2017. Studies involving pregnant women, editorials, pilot studies, conferences, abstracts, thesis, and unpublished work were excluded.
3. THE SOURCE OF BIOMARKERS DISCUSSED HERE ARE
1. Systemic biomarkers, including serum, plasma
2. Ocular biomarkers including tears, vitreous, aqueous
Circulating biomarkers in serum, plasma, and vitreous that are involved in the pathogenesis of a DR are as follows:
3.1. Inflammation
Inflammation plays a significant role in the pathophysiology of DR and contributes to disease development. Increased levels of pro-inflammatory cytokines and chemokines have been found not only in systemic circulation but also in the retina, vitreous humor, aqueous humor, tears, and other ocular fluids. These biomarkers may serve as potential indicators of diabetic retinal disease. The severity of DR has been linked to elevated intraocular inflammatory cytokines and pro-inflammatory cytokines such as interleukin 1, interleukin 6, interleukin 8, hs-CRP, and monocyte chemoattractant protein 1, intracellular adhesion molecule-1, and vascular cell adhesion molecule-1. Notably, the levels of these pro-inflammatory cytokines were found to be lower in patients with proliferative diabetic retinopathy compared to those with non-proliferative retinopathy or no retinopathy [8, 9].
3.2. Tumor Necrosis Factor Alpha (TNF-α)
It is an inflammatory cytokine that is produced and secreted by neurons, muller cells, endothelial cells, and macroglia. The primary sources are smooth muscle cells, endothelium cells, mast cells, β-lymphocytes, T-lymphocytes, neutrophils, and activated macrophages [10]. It increases the formation of reactive oxygen species, which are essential for neovascularization and vasomotor reactions of the retina. Muller cells release TNF-α by activating the EGFR/p38/NF-kβ /p62 pathway. This process leads to apoptosis in retinal pigment epithelial cells and affects the vascular permeability of the retina [11]. Both the apoptotic and nuclear factor-kβ pathways are activated by binding to the two TNF-α receptors (TNFR-I and TNFR-II) found in immune cells [12]. Additionally, TNF-α activates NF-κβ and protein kinase C zeta, which disrupt the tight junctions between individual retinal endothelial cells and retinal pigment epithelial cells [13]. This disruption results in the breakdown of the blood-retinal barrier, but blocking the TNF-α/EGFR axis has been shown to reduce this degradation. Research involving diabetic mice aimed to examine the effects of the TNF-α/EGFR axis on the retinal barrier, with the hope that targeted therapies will be beneficial in treating retinal disease. Similarly, a meta-analysis study on TNF-α in retinopathy indicates a statistically significant difference when compared to healthy controls [14].
3.3. Interleukins-1β (IL-1β)
It can be produced by retinal pigment epithelium and activated glial cells (microglia and macroglia), muller cells, and astrocytes [15]. It has a greater potency for inducing IL-8 expression in muller cells via the p38MAPK and extracellular signal-regulated protein kinase 1 and 2 pathways. This process triggers the production of reactive oxygen species and facilitates the nuclear translocation of NF-kβ, leading to a chronic inflammatory response [16]. The increased expression of IL-1β in DR results in the generation of inflammatory mediators, endothelial cell activation, and stimulation of leukocyte migration. These events make the blood-retinal barrier more permeable and leakier, contributing to retinal edema and neovascularization (Fig. 1) [17].
The release of pro-inflammatory cytokines, along with retinal edema, neuronal death, and vascular leakage, can potentially be mitigated by directly or indirectly inhibiting the NLRP3 inflammasome pathway. Since this pathway primarily functions through the action of IL-1β, it represents a promising therapeutic target for preventing the onset and progression of DR [18].
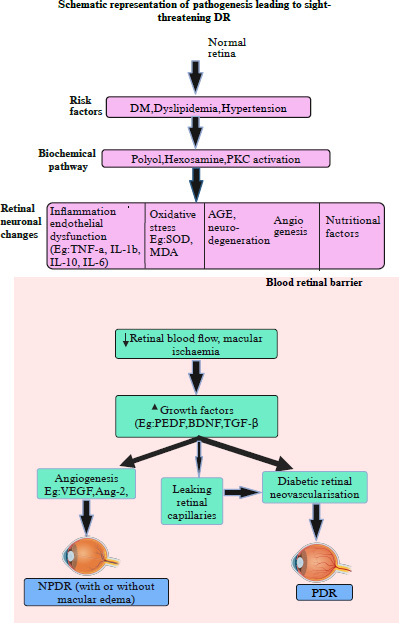
Schematic illustration depicting the pathogenesis of diabetic retinopathy.
3.4. Interleukins-6 (IL-6)
It is a dual-function cytokine linked to the progression of diabetic retinopathy. It plays a role in the synthesis of acute-phase proteins and exhibits a pro-inflammatory effect in chronic inflammation while having an anti-inflammatory effect during acute-phase inflammation. It facilitates the transition from acute to chronic inflammatory conditions by promoting the change in leukocyte infiltration from polymorphonuclear neutrophils to macrophages and monocytes [19]. Additionally, IL-6 activates the Jak-STAT3 pathway via the action of vascular endothelial growth factor, which increases vascular permeability, induces apoptosis, and promotes angiogenesis [20]. It has been linked to early and advanced stages of DR as well as the severity of the disease in DM. Moreover, it was suggested that elevated levels of plasma IL-6 could be used to identify people with diabetes with reduced best-corrected visual acuity. More precisely, readings below 1.85 pg/mL demonstrate a 78% sensitivity and 65% specificity for the diagnosis of significantly deficient visual acuity [21]. Inhibiting IL-6 trans-signaling is considered a promising target for the treatment of diabetic retinopathy, as it can reduce oxidative damage to the retina, address issues related to the endothelial barrier, and decrease vascular inflammation [21]. Furthermore, inflammatory cytokines are found to be significantly higher in the vitreous samples of proliferative diabetic retinopathy compared to non-proliferative diabetic retinopathy [22].
3.5. Interleukins-10 (IL-10)
It is a cytokine synthesis inhibitory factor that possesses anti-inflammatory and anti-angiogenic properties. It also inhibits the production of pro-inflammatory cytokines through immunosuppression [23, 24]. It inhibits the production of pro-inflammatory cytokines through mechanisms of immunosuppression. Research has explored the role of IL-10 in retinal pigment epithelium cells using a model of rhegmatogenous retinal detachment in Wistar rats. The findings indicated that it reduced inflammation, promoted retinal epithelium cell death, and prevented cell proliferation and migration by regulating vascular epithelium growth factor expression [11]. A meta-analysis confirmed that PDR had significantly higher vitreal concentrations of IL-10 than non-DR [25]. However, some studies have found that there is an inverse relationship among the severity of DR [26]. The study by Zhang et al. also confirmed that these levels were significantly higher in the control but decreased in NDR, NPDR, and PDR [27]. Due to these discrepancies, further research is necessary to clarify the roles and underlying mechanisms of IL-10 in the etiology of diabetic retinopathy.
3.6. Advanced glycation end products (AGE)
It is produced when reducing sugars and amine residues on proteins, lipids, or nucleic acids interact nonenzymatically, and its accumulation over time results in cell and tissue damage [28]. In diabetic patients, AGEs are localized in retinal blood vessels, neurons, and glial cells, and their levels correlate with the severity of retinopathy [29]. It typically forms on short-lived proteins in different body tissues, such as albumin, immunoglobulins, hemoglobin, and lipoproteins, as well as long-lived proteins like collagen and crystallin from the ocular lens. When hyperglycemia occurs, AGE products accumulate in the retinas of diabetic patients. This accumulation increases the permeability of retinal endothelial cells, leading to vascular leakage, which is a key factor in the development of diabetic retinopathy. Elevated levels of AGEs in the skin may also predict the degree of DR in diabetic patients [30].
Due to their fluorescent nature, AGEs can be measured non-invasively in the skin, cornea, and lenses using an AGE reader [31]. Additionally, gas chromatography/mass spectrometry or novel slot blot analysis can be used for quantification [32]. Higher serum concentrations of AGEs were found to be associated with microvascular disease, particularly in cases where the patient had retinopathy (85.60±11.66, P≤0.001) [33]. The activation of the TPL2/ATF4/SDF1α pathway by Nε-(carboxymethyl) lysine, a major advanced glycation end product, is a significant factor in endothelial inflammation and neovascularization [34].
When AGEs bind to their receptor, the Receptor for Advanced Glycation End-products (RAGE), they initiate a series of intracellular signaling cascades, including phosphoinositide 3-kinase/protein kinase, B/IκB kinase, and NF-κB activation. These cascades can damage the neural and vascular components of the retina, leading to endothelial dysfunction, vascular inflammation, angiogenesis, pericyte apoptosis, and disruption of the inner blood-retinal barrier [35, 36]. The cumulative effect of these processes results in damage to the neuronal and vascular components of the retina. Promising future treatments may focus on suppressing AGEs/RAGE-mediated downstream signaling cascades and blocking their interactions to reduce this damage [31].
3.7. Oxidative Stress
Increased oxidative stress and reduced antioxidant levels can damage retinal and capillary cells, leading to the development of DR [37]. In addition to being an effect of AGE-RAGE activation, oxidative stress is a strong inducer of RAGE expression. It is constantly produced by all cells to maintain regular cellular processes and to start a series of signaling pathways that cause the inflammatory part of DR. In response to inflammatory cytokines, this involves up-regulating genes and activating NFκβ pathway [38]. It is more commonly formed when AGEs attach to RAGE on endothelial cells' surface, activating NADPH oxidase [39, 40]. Furthermore, oxidative stress triggers the activation of several biochemical pathways, including the hexosamine pathway, the aldose reductase pathway, protein kinase pathways, and the polyol pathway. It was found that the development of DR was facilitated by ORAIP, an oxidative stress-responsive apoptosis-inducing protein. Studies have revealed that levels of vitreous ORAIP are significantly higher in patients with proliferative diabetic retinopathy compared to a control group. Although oxidative stress has been proposed as a mechanism through which ORAIP may damage the retina, further research is required to confirm this theory [41].
3.8. Malondialdehyde (MDA) and Superoxide Dismutase (SOD)
It is a final product of the highly reactive three-carbon dialdehyde and polyunsaturated fatty acid peroxidation in the cells. It is a bifunctional, extremely toxic substance that interacts with DNA to form adducts with deoxyguanosine and deoxyadenosine. Additionally, it alters proteins, RNA, and other biomolecules involved in lipid peroxidation, which in turn interacts with phospholipids in cell membranes [42]. Research indicates that it is elevated in patients with retinopathy compared to healthy controls (Table 1). Meanwhile, SOD is generated as a byproduct of oxygen metabolism and functions as an antioxidant defense. Both MDA and SOD may have potential as effective therapeutic agents against retinal diseases caused by reactive oxygen species [43]. However, further research is needed, as the current data is insufficient to fully support this idea.
3.9. Angiogenesis
These are abnormal blood vessel formations that primarily arise in diabetes. Angiogenic factors are produced more when hyperglycemia and inflammatory mediators are involved in the pathophysiology of DR.
Biomarkers | Groups, no of patients | Main findings | Ref |
---|---|---|---|
1. IL- 1β, and IL-6, IL-8, TNF-α, and VEGF | DR: 14 T2DM but no DR (NDR):16 Healthy Control: 35 |
Serum concentrations of IL-1β, IL-6, IL-8, IL-12, TNF-α, and IFN-γ were significantly higher in the DR group than in the controls. | [44] |
2. TNF-α | Meta-analysis, 1286 participants from 16 paper | No significant difference in patients with DR and healthy group (SD=0.39, 95% CI=−0.09 to 0.68, P = 0.01) but subgroup analysis showed a significant correlation between the level of TNFα and European studies (SMD: 0.57, 95% CI: 0.11–1.02, P = 0.01), with Type 1 diabetes (SMD: 1.06, 95% CI: 0.09–2.04, P = 0.03). | [14] |
Healthy controls:50, NDR:50, NPDR:50 PDR:50 |
Elevated across the severity of diabetic retinopathy. Healthy subject: 3.31 ±0.23 pg/mL. Diabetes without DR:106.74±1.26 pg/mL, NPDR: 22.03± 0.43 pg/mL, PDR:27.32± 0.63 pg/mL, p<0.01. |
[45] | |
3. FGF 21 | Healthy Control:86, NDR:93, DR:91 | Higher in the DR group than without DR and healthy control (P<0.001). In the multivariate logistic regression model, obesity, cholesterol levels, HbA1c, and FGF21 were associated with DR. The best cutoff value for predicting DR was >312 pg/mL. | [46] |
5. VEGF | Healthy: 50 NDR:50, NPDR:50, PDR:50 |
Healthy: 77.38±1.73 pg/ml, DM without DR: 84.91±2.09 pg/ml, NPDR: 90.27±2.11 pg/ml, PDR 106.74±1.26, P = 0.003 | [45] |
NDR:62, NPDR:41, PDR:64 | Controls: 60 pg/mL, NPDR: 133 pg/mL, and PDR: 229 pg/mL and correlated with HbA1c, HDL, and irisin levels. | [47] | |
6. sRAGE, MMP-9, HIF-1a, NO, PEDF, MDA, VEGF-A |
Healthy control:148, T2DM without DR:148 NPDR:148 and PDR:74 |
Plasma cytokines were higher in NPDR and PDR than in no-DR; however, protein thiol levels were lower regardless of disease severity. ROC indicates that the plasma thresholds of HIF-1a (AUC:0.99) and PEDF (AUC:0.90) could be helpful indicators in the case of NPDR. High sensitivity and specificity were demonstrated in PDR by HIF-1a (AUC:1.0), NO (AUC:0.945), MMP-9 (AUC:0.988), and PEDF (AUC:1.0) | [39] |
7. ANGPTL2 | CSME: 48, non-CSME: 55, and DM (control): 69 | CSME group: 4.46 (3.97, 4.96), nCSME-group: 3.80 (3.42, 4.18). DM without DR, control: 3.33 (3.03, 3.63), p<0.01. It is correlated with ME, BCVA, and CRP. | [48] |
8. MDA | DR: 70, T2DM with no DR:40, healthy control:40 | Serum MDA levels were elevated in the diabetic group (with /without DR) than in the control group (non-diabetic). DM Without DR: 12.0±4.99 pg/mL, DM with DR: 18.6±3.67pg/mL. Healthy: 5.47±1.6 pg/mL, P<0.001. | [42] |
10. BDNF | Healthy:20, NDR: 20, NPDR:20, PDR:20 | Plasma BDNF was significantly lower in diabetic patients with DR compared to those without DR. | [49] |
Meta-analysis, 6004 controls and 2734 DM patients |
Compared to controls, DM patients had decreased serum BDNF levels (SMD = -1.00, P<0.001). Subgroup analysis also revealed that serum BDNF levels were lower in diabetes (SMD = -1.26, P<0.001), DM and depression (SMD = -1.69, P<0.001), and patients with DR than in controls (SMD = -1.03, P = 0.01) but there was no apparent variance in plasma BDNF levels between DM patients and controls. | [50] | |
11.GDF-15 | DR:168 NPDR:47 PDR:20 |
The mean levels of plasma GDF-15 varied significantly. NPDR:1114 ng/L, PDR: 1445 ng/L; p for trend = 0.035). With 70.2% specificity and 64.2% sensitivity in predicting DR, the ideal cut-off value was 1336 ng/L. The AUC for GDF-15 was 0.701 (95% CI: 0.629–0.774; p<0.001). |
[51] |
3.10. Vascular Endothelial Growth Factor A (VEGF-A)
It is primarily recognized for its role in the pathophysiology of DR as a pro-angiogenic factor that promotes the formation of new capillaries in the proliferative phase of DR. In addition to retinal pigment epithelial cells, pericytes, Muller cells, astrocytes, and glial cells, endothelial cells are the primary source. . It increases vascular permeability in the hypoxic retina and breaks down the blood-retinal barrier, which both encourage the proliferation and neovascularization of endothelial cells [52]. In addition to promoting the phosphorylation of ZO-1, occludin, and VE-cadherin, which dissolves the blood-retinal barrier, it also increases leukostasis in the microvessels of the retina. Clinical research revealed that PDR patients had significantly higher serum, vitreous, or plasma levels of circulating VEGF than NPDR or no-DR patients [47]. Consequently, a great deal of research has been conducted on medications that either directly or indirectly inhibit the VEGF molecule.
Currently, anti-VEGF medications are regarded as the gold standard treatment for patients with diabetic macular edema. These treatments can improve visual acuity and slow the progression of the condition [53]. The FDA approved the anti-VEGF drug aflibercept as the first-line therapy for newly diagnosed clinically significant macular edema, and it may reduce the frequency of injections needed. The mean visual acuity significantly improved from baseline (47.3 [14.2] vs. 62.2 [13.9] Early Treatment Diabetic Retinopathy Study [ETDRS] letters, P < 0.001) after 12 months of treatment.There were noticeable reductions in central macular thickness, and these reductions persisted throughout the investigation [54, 55]. Other anti-VEGF medications that are commercially available in the market include Avastin (Bevacizumab, Genentech), which has been used off-label for the treatment of several retinal diseases, including DME and is FDA-approved for the treatment of cancer. FDA-approved medication, Lunentis (Ranibizumab, Genentech) for treating Wet AMD, retinal vein occlusion, and DR with or without DME, and Beovu (brolucizumab, Novartis) are used to treat wet AMD [56]. The recommended standard treatment for proliferative diabetic retinopathy is pan-retinal photocoagulation. This laser intervention has been shown to reduce the risk of visual loss by 50%. When combined with anti-VEGF treatment, PRP can help stabilize or even reverse retinal neovascularization [57]. Despite the effectiveness of anti-VEGF injections, there are several drawbacks, including the potential for retinal detachment from intraocular injection, increased treatment costs, adverse side effects, and long-term drug resistance [58, 59]. This prompted the search for non-invasive, alternative therapies.
3.11. Angiopoietins (Ang)
The family of angiopoietins plays a crucial role in regulating the development of new blood vessels. It attaches itself to the important angiogenesis modulators, the Tie1 and Tie2 receptors. It appears that angiopoietin-1 and -2 have both agonistic and antagonistic actions. Ang-1 appears to protect against diabetic damage to the blood-retina barrier and may also have a preventive effect on DR [60]. In contrast, increased expression of Ang-2 leads to the production of pro-inflammatory cytokines, elevated levels of vascular endothelial growth factor, heightened vascular permeability, and retinal neovascularization. These factors contribute to PDR in diabetic patients [61, 62]. A better understanding of the underlying cellular and molecular mechanisms of Angiopoietin-induced endothelial dysfunction can aid in the early detection of DR severity and the development of new anti-angiopoietin medications.
3.12. Angiopoietins-like Proteins (ANGPTL)
It is widely expressed in different tissues, such as the liver, vascular system, and hematopoietic system, and plays a major role in inflammation, lipid metabolism, and angiogenesis [63]. Though ANGPTLs are classified as orphan ligands, because they cannot bind to angiopoietin receptors such as Tie 1, Tie 2, or the related protein, they can still regulate angiogenesis similarly to angiopoietins [64].
3.13. Angiopoietin-like Protein-2 (ANGPTL2)
It is a secretory glycoprotein and a member of the angiopoietin-like family. It is produced by adipose tissue and structurally similar to angiopoietin as it also contains a fibrinogen-like domain and a coiled-coil domain. It is highly expressed in the kidney, lung, skeletal muscle, adipose tissue, and heart [63]. Increased ANGPTL2 generated by endothelial cells and macrophages that invaded the cell stimulated NF-kβ signaling, intensified neovascularization, and endothelial dysfunction by inducing an inflammatory response [48]. According to Yan et al., it is necessary to control angiogenesis, lipid and glucose metabolism, endothelial dysfunction, and chronic inflammation, which regulates inflammatory neovascularization in a mouse model of age-related macular degeneration [65]. Similarly, it also contributes to tissue homeostasis by promoting inflammation and rebuilding via integrin 51/MAPKs and NF-β signaling. There is growing evidence indicating that the overexpression of ANGPTL2 signaling may contribute to chronic inflammation, cancer, and metabolic disorders such as type 2 diabetes [66]. It has also been linked to the severity of diabetic foot ulcers and the onset of diabetes [67]. In a prospective cohort study, elevated serum levels of ANGPTL2 were significantly associated with a higher prevalence of cardiovascular disease [68]. It has a complex role in cell growth; it can either stimulate or inhibit tumor growth depending on the type of cell that produces it. When it is produced by tumor stroma cells, it enhances dendritic cell-mediated responses from CD8+ T cells, which can help inhibit tumors. Conversely, when generated by cancer cells, it promotes tumor growth. This dual role of ANGPTL2 signaling provides new insights into cancer immunity regulation and highlights its complex involvement in cancer pathophysiology [69]. While this protein is involved in the development of retinopathy, its exact physiological and pathological functions remain unclear and need further investigation.
3.14. Angiogenic Markers as a Therapeutic Target
Some individuals develop intolerance to anti-VEGF injections due to their frequent administration, leading to resistance against the treatment. Therefore, it is necessary to develop a topical or oral delivery method as a substitute for invasive intraocular injections. Improvements in diabetic retinopathy severity, specifically in patients with non-proliferative diabetic retinopathy, can potentially be achieved by using a Tie-2 activator to target the Ang-2 pathway. This claim is supported by a study that shows a significant reduction in diabetic macular edema when comparing anti-VEGF monotherapy to AKB-9778 [70].
3.15. Neurotransmitter and Neuroprotective Factors
Neurotransmitters and neuroprotective factors have a negative impact on the retina during hyperglycemia. Furthermore, neurodegeneration leads to hypoxia, vaso-regression, and the disruption of the blood-retinal barrier, all of which are significant indicators of neovascularization.
3.16. Brain-derived Neurotrophic Factor (BDNF)
It is a neurotrophic factor that supports the growth of pancreatic β cells and contributes to the survival and plasticity of neurons by acting as a neurotransmitter modulator [71]. It binds to the high-affinity receptor tyrosine kinase B, activating the IRS1/2, PI3K, and Akt signaling pathways. This activation promotes the production of genes that code for proteins essential for cell life, helping β cells to thrive. Additionally, BDNF plays a role in regulating glucose and energy metabolism, thus preventing the deterioration of β cells. Conversely, reduced levels can lead to poor glucose regulation [50]. It may aid in the prevention or treatment of type 2 diabetes in addition to its potential advantages for enhancing insulin sensitivity, increasing energy expenditure, and regulating blood glucose levels. Low levels of LXA4 and BDNF, which are important for controlling pro-inflammatory and anti-inflammatory cytokines, have been linked to the development of diabetic retinopathy [72, 73].
3.17. Pigment Epithelium-Derived Factor (PEDF) or Serpin Family F Member 1 (SERPINE 1)
It was first identified in the retinal pigment epithelium and is now known to be extensively distributed in the body. This protein is encoded by the SERPINE 1 gene. The primary sources are the liver and adipocytes, but it is also found in the kidney and arteries. It supports the survival of photoreceptors, retinal progenitor cells, and retinal neurons in the eyes by having a neurotrophic effect [74]. Additionally, this protein possesses anti-inflammatory, antioxidant, anti-angiogenic, and neuroprotective properties. It achieves these effects by blocking the activation of specific pathways, including NF-κB and ERK1 [75, 76]. Furthermore, it protects against both glutamate excitotoxicity and oxidative stress. It is referred to as an “angiogenic inhibitor” because it functions as an antagonist to VEGF, which may suppress ischemia-induced retinal neovascularization and inhibit retinal endothelial cell proliferation and migration [76]. Currently, potential alternatives to existing anti-VEGF therapies, such as pigment epithelium-derived factor, cell therapy-based blocking of transforming growth factor beta, and anti-platelet-derived growth factor-BB, are being investigated [77].
3.18. Fibroblast Growth Factor 21 (FGF-21)
It has proangiogenic properties and is primarily produced by the liver, with trace amounts found in skeletal muscles, pancreatic β-cells, and adipose tissues. It may serve as a compensatory mechanism for metabolic dysregulation [78]. It has been found that FGF-21 binds to its receptor and forms a complex with β-klotho, which enables it to physiologically regulate the gluconeogenesis and lipolysis of the liver and adipose tissues during fasting and starvation [79, 80]. Higher levels have also been associated with insulin resistance, obesity, hypertension, diabetes, and cardiovascular disease [81]. In a cross-sectional study conducted by Heidari Z, Hasanpour M., significant correlations were observed between FGF21 levels and the duration of diabetes mellitus and triglyceride levels in diabetic patients with retinopathy [46].
3.19. Growth Differentiation Factor-15 (GDF-15)
It is also known as prostate-derived factor, placental transformation growth factor, macrophage inhibitory cytokine-1, and non-steroid anti-inflammatory drug activated gene-1. It exhibits high expression in adipocytes, cardiomyocytes, macrophages, endothelial cells, and vascular smooth muscle cells in both healthy and pathological conditions, and it is a member of a distinct subfamily of TGF-β [82]. In patients with type 2 diabetes, a positive correlation has been observed between serum GDF-15 levels and complications from micro- and macrovascular diseases (OR: 1.40) [83]. Research conducted by Adela and Frimodt indicated that its expression reduces the formation of the NLRP3 inflammasome, which is associated with insulin resistance in obesity [82]. Recent studies have focused on GDF-15 as a potential therapeutic target for weight control, particularly due to the discovery of its receptor, GFRAL, which is expressed in the brainstem of humans, mice, and primates [84]. Higher circulating levels were also associated with the severity of diabetic retinopathy [85]. Additionally, it serves as a valuable biomarker for predicting cardiovascular events and mortality. It aids in diagnosing patients with non-ST-elevation acute coronary syndrome and helps facilitate referrals for early revascularization and more intensive care [86]. The role of plasma GDF-15 was examined in patients with established CAD to predict long-term outcomes, including major adverse cardiac events and all-cause mortality in a large cohort [87]. Further investigation is necessary to determine its potential therapeutic impact and its functional role in retinal pigment epithelial cells.
3.20. Tumor Growth Factor (TGF-β)
It is a proinflammatory cytokine involved in cell motility, proliferation, and differentiation and the synthesis and degradation of extracellular matrix components. When TGF signaling is dysregulated, it leads to an increased production of ECM components, resulting in the thickening of the basal membrane [88]. It also promotes antioxidant and neuroprotective pathways through the Nrf2/Keap1/ALDH3A1/HO-1 signaling pathway, which helps prevent oxidative damage to retinal ganglion cells caused by hyperglycemia. The TGF-triggered antioxidant signaling pathway has been connected to stress response proteins such as heme oxygenase-1, aldehyde dehydrogenase 3A1, Keap 1, nuclear factor erythroid 2-related factor, and hypoxia-inducible factor [89]. By stimulating the signaling pathway, it may act as a potential therapeutic target for retinal disease. Additionally, it works in conjunction with VEGF to promote angiogenesis and remodel the extracellular matrix in cases of retinal fibrosis [90].
A study by Bonfiglio investigated the effects of drugs on these markers, specifically focusing on eyes treated with aflibercept. The findings revealed that patients with NPDR who received aflibercept treatment had higher serum levels of TGF compared to those with diabetes but without retinopathy. This suggests that measuring VEGF-A and TGF-β1 levels could serve as a secondary endpoint for assessing the clinical efficacy of anti-VEGF treatments and may act as a predictive biomarker for the progression of non-proliferative diabetic retinopathy (Table 2) [91].
Biomarkers | Methodology | Main findings | Ref |
---|---|---|---|
1.IL-1β, Il-6, IL-8, TNF-α, NGF, BDNF, NT-3, NT-4, CNTF, GDNF (Vitreous) | DR:22 Non diabetic (control):28 |
All cytokines are higher in the DR than in the control, but TNF-α (P < 0.05), IL-8 (P < 0.004), NT-3 (P = 0.012), NGF (P = 0.04), GDNF (P = 0.005), and CNTF (P = 0.002), were higher in eyes with NPDR than PDR. | [92] |
2. VEGF, SDF-1α, and ANGPTL2 (Vitreous) | PDR: 31 and idiopathic macular hole (control): 10 | Significantly higher in eyes with active PDR than in the idiopathic macular hole. | [93] |
3.LCN2, IL6, IL8, IL1β, IL27, ICAM, VCAM-1, MCP-1, TNF-α, and VEGF-A (Vitreous) |
Control with vitreomacular interface pathology such as iERM, VMT, macular hole: 11, PDR: 13, DME:7 | All cytokines are higher in the PDR than in the control, and LCN2, IL6, IL8, IL27, ICAM, VCAM-1, TNF-α, and VEGF-A were higher in DME patients than in controls. | [22] |
4.IL-6, IL-8, VEGF, PlGF (Aqueous) |
98 DME patients divided into 43 NPDR, 17 PDR, and 38 PRP | VEGF and PIGF were significantly higher in the PDR group than in the NPDR and PRP group, but TNF-α level increased early after PRP. | [94] |
5. IL-1β, IL-6, IL-8, IL-17, and TNF-α (Vitreous) |
Without DR:40 With DR:40 |
Compared with different PDR stages of retinal neovascularization. Based on diabetes duration, these cytokines were higher (p < 0.05) in the 5-year PDR group and the 10-year PDR group than in the 5-year NPDR and the 10-year NPDR. |
[9] |
6. IL1β, IL-6, IFN-γ, IL-2, IL-4, IL-13, VEGF, VEGF-A, PGF. (Vitreous) | Macular hole (Control):17, DR:17 | Upregulated: IL-1β, IFN-γ, VEGF, PGF Comparable: IL-6, IL-2, IL-4 Downregulated: IL-13 |
[95] |
7. IL-23, IL-17, IL-10 and TGF-β. (Aqueous) |
Control:20, NDR:18, NPDR:17, PDR:15 | IL-23 level was higher in the NDR group and raised along with the DR progression. IL-17 level was higher in NPDR and PDR than in NDR control. By contrast, IL-10 was lower in DR than in non-diabetics and decreased as the severity of DR increased. TGF- β was also elevated in diabetes but showed no differences based on the presence or severity of DR. | [27] |
8. IL-6, IL-8, IP-10, LIF, VEGF, IL-1α, IL-4, IL-9, IL-21, IL-23, IL-27, IL-31, RANTES, interferon-α, GRO, TNF-α. (Aqueous) |
NDR:50, NPDR:49, PDR:52, non-diabetic: 51. | IL-6, IL-8, IP-10, LIF, HGF, and VEGF-A were significantly higher in DR than in nondiabetic, and remaining cytokines were significantly lower in DR. IL-6 were positively correlated with the IL-8, HGF, and LIF levels and negatively with the IL-31and GRO levels. | [26] |
9. IL- 1β, IL-2, IL-4, IL-5, IL-6, IL-10, IFN-γ, TNF-α, VEGF. (Aqueous) |
Control (no T2DM): 10 NDR: 22, NPDR: 15 PDR: 14 |
No difference was observed in the aqueous concentrations of all cytokines between the control and the NDR group but it significantly increased with the severity of DR. | [96] |
10. VEGF, IL-6, ANG-1, ANG-2, UPAR, leptin, Follistatin, MMP-9, PIGF, ANG (Vitreous) | PDR: 10, Non-diabetic (control): 9 | Cytokines were significantly increased in PDR but Angiogenin and Leptin were significantly lower in PDR eyes. | [97] |
11. IL-6, IL-8, IP-10 and MCP-1, VEGF (Aqueous) | Nondiabetic:37, NDR:13, early, mod NPDR:12 | Cytokines are significantly higher in NPDR groups than in no-DR or non-diabetic but have no significant correlation with IOP, fasting blood sugar, HbA1C, or CRP. With an AUC of 0.897 (p = 0.001, 95% CI = 0.74–1.0). | [98] |
12. IL-1β, IL-8, MCP-1 and VEGF (Aqueous) | Control: 35, DR:14, Without DR: 16 |
IL-1β, IL-8, MCP-1, and VEGF were significantly higher in DR as compared with the NDR. | [44] |
13. VEGF, PIGF, TGF-b, IL-6, IL-8, IL-10, vascular cell adhesion molecule-1, MCP-1 (Aqueous) |
49 DME patients with a severity of NPDR | All cytokines were associated with disease severity. ICAM-1 was correlated with greater macular volume (P = 0.002). IL-10 levels with best-corrected Snellen visual acuity (P = 0.03). No aqueous cytokine, including VEGF, correlated with central subfield macular thickness. | [99] |
14. TGF-β1, TGF-β2, TGF-β3, MMP-3, MMP-9, and TIMP-1 (Aqueous) |
Healthy control: 17, NDR:23, mild/ mod NPDR:13, advanced NPDR/ PDR without vitreal hemorrhage:14 | TGF-β1, MMP-3, and TIMP-1 levels were increased in PDR than non-diabetic. | [91] |
15. BDNF (Aqueous) |
Healthy:30 NDR:30 NPDR:30, PDR:30 |
BDNF level is lowered in all groups than control. Not correlated with DM duration (P = 0.522) or HbA1C (P = 0.862). | [100] |
3.21. Neuropeptide as a Therapeutic Target
Neuropeptides are small proteins that have a neuroprotective effect on retinal diseases and function as signaling molecules in the nervous system. It has been found that neuropeptides are involved in neuromodulation and the processing of visual information by the retina. In general, neuropeptides can regulate extracellular glutamate, which supports the survival of retinal neurons and may also possess anti-inflammatory or antioxidant properties [101]. Peptidergic substances generated in the retina, along with their receptors, have neuroprotective effects in several retinal disease models and it may open the door for novel pharmacological strategies. According to Cai, studies on the mRNA and protein levels of Glucagon-like peptide 1 and GLP-1R in the retinas of rats, mice, and humans have demonstrated neuroprotective effects that may prevent retinal neurodegeneration. Furthermore, the study stated that in the context of diabetic retinopathy, GLP-1 therapy regulates autophagy by inhibiting the expression of HDAC6, a protein implicated in oxidative stress, through the GLP-1R-ERK1/2-HDAC6 signaling pathway [102]. GLP-1R agonists like exendin -4 and liraglutide have been demonstrated to shield retinal cells from oxidative stress-induced damage, while NAP promotes neurite outgrowth in retinal ganglion cells [103].
In a study conducted on mice with 24 weeks of chronic diabetes, it was discovered that topical application of GLP-1 not only reverses these important pathogenic processes linked to DR but also prevents neurodegeneration and vascular leakage from advancing. The downregulation of VEGF and its anti-inflammatory effect are the primary processes involved. Its role in neurogenesis suggests that it may act as a therapeutic target for retinal neurodegenerative disorder. At prescribed dosages, systemic administration of these neuropeptides is unlikely to reach the retina and may have unfavorable effects on the system [104]. Due to the low bioavailability of peptidergic substances, the peptide-based therapeutic approach is still in the pre-clinical stage.
4. NUTRITIONAL FACTORS
Food supplementation with vitamins, minerals, and nutraceuticals is highly recommended for micro- and macrovascular complications as it is simple, inexpensive, and easily available. Genetic variations and dietary deficiencies usually significantly impact complex systems with an abundance of cofactors and substrates [105]. Multivitamins, mineral complexes, and B-complex multivitamins have long been used to treat and prevent various nutritional deficiencies because mono-deficiencies are uncommon [106]. Although retinopathy is supported mechanistically by such vitamin-antioxidant combinations, only a few studies have demonstrated the effectiveness of nutraceuticals in the clinical approach. The processes of angiogenesis, inflammation, and fibrosis, which are the root cause and mechanism of DR, are significantly influenced by vitamin A. It is widely known that retinol, another name for vitamin A, is a part of the pigment in the eye called rhodopsin, which is sensitive to light. Additionally, the integrity of the conjunctival and corneal membranes must be maintained otherwise, its deficiency leads to retinal impairment. In another study, serum vitamin A levels were raised by 31.1%, which decreased the risk of diabetic retinopathy [107].
Circulating levels of retinol-binding protein 4 have been associated with several metabolic conditions, including insulin resistance, metabolic syndrome, impaired glucose tolerance, and type 2 diabetes [108]. The relationship between DR and retinol-binding protein was examined in a study, which revealed that patients diagnosed with DR exhibited significantly elevated concentrations compared to those without DR, with values of 39.7 (interquartile range: 29.8–47.7) μg/ml versus 25.0 (IR range: 15.5–32.6) μg/ml, respectively (P < 0.001) [109].
The blood-retina barrier and blood-brain barrier are easily crossed by two plants based, and water-solublecarotenoids called lutein and zeaxanthin. Although the human body is unable to produce them, they are vital for vision. They serve as a strong antioxidant that protects cell membranes from oxidative damage and are concentrated in the macula lutea. They also offer defense against DR and AMD. Patients with diabetes are found to benefit from antioxidants, particularly those containing carotenoids and vitamins C and E. It protects against oxidative stress, stabilizes cell membranes, and lessens endothelial dysfunction [110]. PDR patients have ten times lower vitreous humor ascorbate levels and are more likely to develop diabetic macular edema [111]. In Gurrieri's Dose-dependent study, vitamin C alone with statins lowers nonproliferative diabetic kidney disease [112]. Following vitamin E therapy, DR symptoms are lessened [113]. It has been discovered that sustaining ideal vitamin D levels can reduce the risk and severity of DR [114]. The function of the β cells in the pancreas depends on the deficiency of vitamin D that raises the risk of T2DM, hypertension, atherosclerosis, and cardiovascular disease, as well as lowering insulin sensitivity. To reduce inflammation, increase insulin sensitivity, and lessen vascular stiffness, one must have adequate levels of vitamin D [115].
Polyphenol is the most common antioxidant found in our body. Nonflavonoid polyphenols like resveratrol and curcumin have anti-inflammatory and antioxidant properties and help prevent retinal neurodegeneration [116]. The flavonoid polyphenol hesperetin inhibits VEGF expression and prevents retinal thinning in diabetic retinas, favoring the preservation of ganglion cells. Additionally, it lessens the expression of caspase-3, which prevents neuron death [117]. Similar to this, naringenin also exhibits neuroprotective effects by encouraging an increase in BDNF and synaptophysin, along with a decrease in apoptotic levels [118]. A prospective multicentre randomized clinical trial called age-related eye disease studies was conducted to evaluate the effects of antioxidant vitamins, minerals, and micronutrients in treating macular degeneration of the eye. These micronutrients reduced the amount of acellular retinal capillaries that accumulated, and a positive correlation was observed between them with supplements containing ascorbic acid, vitamin E, β-carotene, zinc, copper, lutein/zeaxanthin, and progression of retinal disease such as AMD [119].
Deficiency in essential vitamins and minerals is common in DR patients, which elevates homocysteine levels and results in oxidative stress. Elevated homocysteine is associated with reduced cerebral blood flow, retinal blood flow, decreased central retinal artery caliber, VEGF production, and diabetic reticulum injury [108]. Structural loss of neurons, photoreceptors, and capillary endothelium happens after initial impairment of Müller cells and mitochondrial dysfunction. Microaneurysms, exudates, cotton wool patches, capillary dropout, retinal edema, and retinal atrophy are signs of this process occurring in the eye [120].
In the context of hyperglycemia, the expression of MMP is altered. This endopeptidase plays an important role in the emergence of numerous visual disorders and is also extensively expressed in ocular tissue [121]. It is generally accepted that the pathological alteration of DR is due to the changes in vascular permeability caused by increased MMPs in the retina that break down the extracellular matrix in the basement membrane of the microvessels. However, the precise mechanism underlying these changes is unknown. Current studies have found that levels of MMP-1, MMP-7, MMP-9, and MMP-14 are higher in the vitreous of PDR compared with control [122, 123]. While nutrition is often considered a crucial factor in managing type 2 diabetes and its complications, there is currently no scientific evidence to support this idea. Therefore, it is essential to develop comprehensive dietary guidelines aimed at preventing the onset of diabetes and its related diseases. Future research and additional experimental models are necessary to better understand the impact of key dietary components on the progression of DR.
4.1. Limitation
It has been found that DR is connected to miRNAs as they act as a significant regulator of particular pathogenic features of DR in recent years. The consequences of circulating mRNA and microRNA were not reviewed here because there is a plethora of publications on that topic.
As there are not sufficient studies that support DR in Type 1 DM, it is not included.
CONCLUSION
Currently, there are only a limited number of studies investigating the potential application of biomarkers in clinical trials. These biomarkers, which are implicated in the pathogenesis of diseases, may facilitate earlier diagnosis, enable monitoring of disease progression, and support therapeutic interventions. However, their practical implementation necessitates validation through long-term studies. It is essential to analyze the relationship between these biomarkers and ocular parameters such as intraocular pressure, visual acuity, refractive error, and optical coherence tomography metrics. Such examinations will enhance our understanding of visual function and facilitate the identification of individuals at higher risk of irreversible vision loss at an early stage. Ultimately, this could improve patients' quality of life by reducing the impact of vision loss and alleviating the overall burden of the disease.
AUTHORS’ CONTRIBUTION
The authors confirm their contribution to the paper as follows: validation: RA; draft manuscript: ST, LC. All authors reviewed the results and approved the final version of the manuscript.
LIST OF ABBREVIATIONS
MDA | = Malondialdehyde |
SOD | = Superoxide Dismutase |
FGF | = Fibroblast Growth Factor, |
GLP-1 | = Glucagon-Like Peptide 1 |
MMP | = Matrix Metalloproteinase |
IL- 1β | = Interleukins-1β |
IL- 1β | = Interleukins-1β |
IL-6 | = Interleukins 6 |
IL-8 | = Interleukins 8 |
TNF-α | = Tumor Necrosis Factor-Alpha |
Vegf | = Vascular Endothelial Growth Factor |
FGF 21 | = Fibroblast Growth Factor 21 |
PEDF | = Pigment Epithelium-Derived Factor |
ANGPTL2 | = Angiopoietin-like protein-2 |
BDNF | = Brain-derived neurotrophic factor |
GDF-15 | = Growth differentiation factor-15 |
SDF-1α | = stromal cell-derived factor |
GNDF | = Glial cell line-derived neurotrophic factor |
IFN-γ | = Interferon-gamma |
IL-4 | = Interleukins 4 |
PIGF | = Placental Growth Factor |
FGF 21 | = Fibroblast Growth Factor 21 |
ICAM | = Intercellular Adhesion Molecule-1 |
VCAM-1 | = Vascular Cell Adhesion Molecule |
MCP-1 | = Monocyte Chemoattractant Protein |
sRAGE | = Soluble Receptor for Advanced Glycation end products |
ACKNOWLEDGEMENTS
Declared none.