All published articles of this journal are available on ScienceDirect.
New Horizons in the Study of WNT and JAK/STAT Signaling Pathways in Cardiometabolic Diseases
Abstract
Aim
Despite advances in diagnosis and treatment, cardiometabolic diseases remain a major worldwide health problem. A new direction in identifying biomarkers that increase diagnostics predictive potential is the study of the WNT and JAK/STAT signaling pathways. Experimental and clinical studies have provided mixed evidence that determined the purpose of the study. This study aimed to examine the characteristics of the production of certain JAK/STAT and WNT signaling proteins in cardiometabolic pathology patients.
Materials and Methods
The research involved patients with myocardial infarction and cardiometabolic syndrome, as well as healthy individuals. Measurement of proteins STAT-1, STAT-3, STAT-6, β-catenin, sclerostin, WIF-1, GSK-3 α, and β, DVL-1 serum concentrations was carried out by ELISA.
Results
We established a wide range of JAK/STAT and WNT signaling protein values in the patient’s blood serum. In cardiometabolic syndrome, there was an increase in the concentrations of β-catenin, DVL-1, GSK-3α, and GSK-3β and a decrease in STAT-1, 3, compared with healthy individuals. During myocardial infarction, an increase in β-catenin, WIF-1, and DVL-1 and a decrease in sclerostin, GSK-3α, STAT-1, STAT-3, and STAT-6 were recorded compared with healthy individuals. The most significant intergroup differences were found for β-catenin, WIF -1, DVL-1, GSK-3α and STAT-6. Statistically significant correlations between the levels of a number of JAK/STAT and WNT signaling proteins and lipid profile parameters were revealed.
Conclusion
The data received about changes in the production of proteins of the WNT and JAK/STAT signaling pathways expand the molecular mechanisms of cardiometabolic diseases' immunopathogenesis understanding.
1. INTRODUCTION
Over the past ten years, the problem of cardiometabolic diseases, including diabetes, stroke, and Coronary Heart Disease (CHD), has become increasingly relevant and medically important throughout the world due to the high prevalence and mortality rate.
Over the past 30 years, mortality from Cardiovascular Diseases (CVD) has increased by 60% worldwide, from 12.1 million in 1990 to 20.5 million in 2021. In 2022, CVD, mainly stroke and CHD, were the main cause of death and disability in the world [1-3].
In 2013, the United Nations set a goal of reducing premature mortality from CVD by 25% by 2025, with a particular focus on reducing cardiometabolic, behavioral, environmental, and social risk factors [4].
In recent years, significant progress in cardiovascular risk assessment has been achieved through an increase in the number and variety of biometric monitoring technologies. It became possible due to the development and widespread use of molecular diagnostic methods. Today, there is an active search for informative biomarkers to assess the risk of occurrence, development, and unfavorable prognosis of cardiovascular pathology.
The last two decades witnessed a major revolution in the field of immunology, the technological advances of which paved the way for a deeper and more complete understanding of immune processes and inflammation of the myocardium and blood vessels [5-8] which significantly changed the understanding of the pathophysiological mechanisms of cardiometabolic diseases.
However, until recently, immunological issues related to cardiology were considered interdisciplinary, and only in recent years has cardiac immunology come into the spotlight, uniting disparate fields.
A new direction in the study of biomarkers that increase the predictive potential of diagnosis is the study of the signaling pathways WNT, JAK/STAT, involved in the pathogenesis of cardiometabolic syndrome (CMS) and CVD [9-13].
In this regard, the goal of the research is to study the characteristics of the production of certain JAK/STAT and WNT signaling proteins in patients with cardiometabolic pathology.
2. MATERIALS AND METHODS
2.1. Subjects of Research
A prospective cross-sectional cohort study was conducted on the basis of the cardiology departments of the Orel Regional Cardiovascular Center (Russian Federation).
The research involved 78 young and middle-aged adults (from 18 to 59 years old, according to the WHO) and 50 healthy individuals (HI) matched by age and gender.
We divided all subjects into 3 groups:
Group I – HI (control, average age – 48.8±5.4 years old) with normal body weight.
Group II – patients with CMS who have a high risk of developing myocardial infarction (38 people, average age – 45.9±7.7 years).
Group III – patients with acute myocardial infarction (MI) (40 adults, average age – 50.8±7.4 years).
No statistical differences were found according to the age (p>0.05).
In our research, the diagnosis of patients with acute MI and metabolic syndrome was established according to the clinical recommendations of the Ministry of Health of the Russian Federation in the current edition at the time of the study.
2.2. Ethics Statement
The study was conducted in accordance with Goоd Clinical Practice standards and the principles of the Helsinki Declaration, the Ethics Committee of the Orel State University named after I.S. Turgenev (Protocol No.17 of December 10, 2019). All patients signed an informed consent form for the use of their examination results for scientific purposes. For each patient, an electronic card was developed and filled out, including personal data, anamnesis of life and illness, physical examination data, and results of laboratory and instrumental examination. All patients involved in the study were assigned a digital code. Interpretation and processing of the results were presented anonymously.
2.2.1. Criteria for Inclusion in the Study
Patients with established diagnosis of CMS and acute MI (young and middle-aged adults according to the WHO).
2.2.2. Criteria for Non-inclusion in the Study
Age under 18 years old and over 59 years old; cardiogenic shock; hypertension above grade 2; chronic heart failure above stage I; verified oncological, autoimmune, mental, chronic obstructive pulmonary diseases; lipid metabolism pathology; decompensated diabetes mellitus; liver cirrhosis; exacerbation of the stomach and duodenum peptic ulcer; pathologies of hemostasis; pregnancy and lactation; acute infectious diseases.
2.4. Sample Collection
The scope of clinical studies included a definition of general blood test indicators, dynamic troponin levels, lipid profile, C-reactive protein, electrolytes (potassium, sodium), glucose. General clinical laboratory tests were performed using “Maxm Analyzer” (UK) and “Monarch chemistry system” (Italy).
Venous blood sampling (15 ml) was taken in the morning in the first 24 hours from the moment of hospitalization, and then the blood was centrifuged for 15 minutes at a speed of 1500 rpm, the serum was aliquoted, frozen at -24 degrees Celsius and stored for 1- 2 months without repeated defrost cycles before use.
Elevation was defined as a serum value greater than the upper limit of normal according to clinical laboratory standards.
2.5. Enzyme-linked Immunosorbent Assay
The studied biomarkers were determined in duplicate samples using enzyme-linked immunosorbent assay (ELISA). Optical density measurements were carried out using a STAT FAX 2100 photometer (Awareness Technology, USA).
To assess the serum concentrations of proteins STAT-1, STAT-3, and STAT-6, test systems by Cusabio Biotech Co. (USA) were used. WNT-signaling pathway (β-catenin, sclerostin, WIF-1, GSK-3α, and β, DVL-1) by Sunlong Biotech Co. (China) reagent kit (sensitivity of 0.5 pg/ml), according to the instruction manual, were used.
2.6. Electrocardiogram
Electrocardiogram (ECG) recording was carried out according to the generally accepted method in 12 leads on device ECG-9803 Medinova Industrial Co., Ltd.
2.7. Body Mass Index
Body mass index (BMI) was determined using the Quetelet method. The degree of obesity was determined according to the WHO classification.
2.8. Statistical Analysis
Statistical analysis was performede by StatTech 3.1.10 program (Russia, 2023).
Shapiro-Wilk test was used to assess quantitative indicators for compliance with a normal distribution (if the patients’ number was less than 50).
Data are presented as the absolute number (%) of patients, as mean value and mean absolute error (M±m). In the absence of normal distribution, quantitative data were described using the median (Me) and the first and the third quartiles (Q1; Q3).
Student's t-test was used for the comparison of two groups according to the quantitative indicator with normal distribution on condition of equality variances; Mann–Whitney U test – for the comparison of two groups according to the quantitative indicator with abnormal distribution.
Kruskal–Wallis test was used for the comparison of three groups, and Dunn's test with Holm's correction was used for post hoc comparison.
Spearman's rank correlation coefficient was used to assess the direction and strength of the correlation between two quantitative indicators (if the distribution of indicators was abnormal). Pearson correlation coefficient was used to assess the direction and strength of the correlation between two quantitative indicators(with normal distribution).
The Chaddock scale is used to interpret correlation strength between two quantitative indicators.
All statistical comparisons were two-sided, and p<0.05 was considered significant.
To assess the diagnostic significance of quantitative characteristics in predicting a specific outcome, the ROC curve method was used.
3. RESULTS
3.1. Clinical Characteristics of Study Participants
Demographic and clinical characteristics of patients in the study groups are presented in Table 1.
Parameter | Group I (n = 50) | Group II (n = 38) | Group III (n = 40) |
---|---|---|---|
Age (years old) | 48.8±5.4 | 45.9±7.7 | 50.8±7.4 |
Gender male/female (%) | 76.0/24.0 | 73.7/26.3 | 80.0/20.0 |
BMI (kg/m2) | 20.9±2.2 | 34.0±2.9* | 29.8±5.5* |
SBP (mmHg) | 119.8±7.7 | 143.6±11.5* | 130.5±15.5* |
DBP (mmHg) | 71.1±5.8 | 92.4±10.7* | 85.4±6.5* |
Glucose (µmol/L) | 4.8±0.6 | 5.3±0.7 | 6.7 (3.5; 12.1)*/** |
Hb (g/L) | 133.6±16.4 | 133.4±9.5 | 154.8±13.4* |
RBC (×10*12/L) | 4.4±0.4 | 4.4±0.5 | 4.9±0.5 |
WBC (×10*9/L) | 5.9±1.2 | 7.1±1.2 | 11.5 (6.1; 41.0)* |
ESR (mm/hour) | 6.1±4.1 | 14.0±6.4* | 15.2 (5.1; 40.9)* |
Cholesterol (mmol/L) | 4.5 ±0.5 | 5.6±0.6* | 5.3±1.5* |
Total bilirubin (µmol/L) | 12.7±4.6 | 18.0±6.5 | 15.1±7.4 |
ALT (IU/L) | 17.0±7.7 | 31.3±9.7* | 51.6±21.4* |
AST (IU/L) | 17.6±5.8 | 30.6±9.4* | 86.5 (30.1; 270.2)* |
Total protein (g/L) | 70.8±3.9 | 68.4±5.4 | 71.7±6.9 |
ALP (IU/L) | 65.4±12.4 | 74.1±21.0 | 151.6 (81; 249.0)* |
Creatinine (µmol/L) | 74.3±12.6 | 71.8±12.1 | 85.8±16.5 |
TG (mmol/L) | 0.85±0.2 | 1.6±0.5* | 1.2±0.4*/** |
HDL (mmol/L) | 1.4±0.1 | 1.2±0.1 | 1.3±0.2 |
LDL (mmol/L) | 1.7±0.2 | 3.1±0.4* | 3.4±1.3* |
VLDL (mmol/L) | 0.47±0.1 | 1.7±0.6* | 0.6±0.2** |
Sodium (mmol/L) | 133.1±2.1 | 128.1±13.3 | 138.1±3.3 |
Potassium (mmol/L) | 4.2±0.2 | 4.1±1.1 | 4.49±0.3 |
Abbreviations: BMI - body mass index, Hb –hemoglobin, RBC - red blood cells, WBC - white blood cells, SBP - systolic blood pressure, DBP - diastolic blood pressure, ESR - erythrocyte sedimentation rate, ALT - alanine aminotransferase, AST - aspartate aminotransferase, HDL - high-density lipoproteins, LDL - low-density lipoproteins, VLDL - very low-density lipoproteins, TG – triglycerides, ALP – alkaline phosphatase.
There were no significant differences in the patients of all groups by age, gender, RBC, bilirubin levels, total protein, creatinine, HDL, VLDL, sodium, and potassium. At the same time, the levels of RBC, bilirubin, total protein, ALP, creatinine, HDL, sodium and potassium were within normal limits for all groups.
The indicators of BMI, SBP, DBP, cholesterol, glucose, WBC, ESR, ALT, AST, and LDL in patients with myocardial infarction exceeded the reference values and had significant differences compared to HI (p<0.05).
In patients with CMS, the values of BMI, SBP, DBP, cholesterol, ALT, AST, TG, LDL, VLDL were significantly higher than in HI (p<0.05).
In addition, we found statistically significant intergroup differences in patients of groups II and III according to levels of glucose, TG, and VLDL (p<0.05). The higher lipid profiles (TG, VLDL) in patients with CMS, which is apparently associated with the identified lower adherence to therapy with lipid-lowering drugs than in patients with MI (42.1% and 70.0%, respectively), are of great importance.
3.2. ECG Results
MI with ST-segment elevation was observed in 35% (n=15) of patients in group III; transmural myocardial infarction was typical for 42.5% (n=17) of patients.
Changes in the ECG in patients with CMS were characterized by minor deviations (in the form of supraventricular extrasystole, flattening of the T wave) in 42.1% of cases (n=16), normal ECG was recorded in 57.9% (n=22) of group II people.
All patients in group I had no changes in the ECG.
3.3. Analysis Results in WNT Signaling Proteins
Considering the important role of the WNT signaling pathway in cardioembryogenesis [11, 14], serum concentrations of the morphogenic WNT signaling proteins (β-catenin, sclerostin GSK-3α, GSK-3β, WIF-1 and DVL-1) were studied by ELISA in acute MI, cardiometabolic syndrome, HI. The obtained data are presented in Table 2.
The results of key mediators of the WNT pathway β-catenin studies showed that patients of all groups had a wide variability of its values in the blood serum. A significant increase in the concentration of β-catenin was revealed in patients of groups II and III, compared with healthy controls. In addition, the increase in β-catenin concentration in patients of group II was most pronounced with BMI>35 kg/m2 and significantly correlated with the degree of obesity (r = 0.554, p<0.001).
When increasing the concentration of β-catenin in the blood serum, we observed a significant increase in the levels of TG, cholesterol, LDL, and VLDL. The high positive correlation between β-catenin and VLDL in patients of group II (r = 0.750; p<0.001) is of great importance. The most pronounced disorders of the lipid spectrum were recorded in patients of group III with a β-catenin value above 400 pg/ml. Fig. (1) shows the ROC curve of β-catenin levels in patients with MI and hyperlipidemia or without hyperlipidemia (area under the ROC curve – 0.744±0.088 with 95% confidence interval: 0.571–0.917; p=0.018).
Some data have been published recently about the sclerostin role in atherosclerosis pathogenesis. However, the results obtained on determining serum concentrations in patients with cardiovascular pathology are few and contradictory [15, 16].
Parameter, pg/ml | Group I (n = 50) | Group II (n = 38) | Group III (n = 40) |
---|---|---|---|
β-catenin | 63.5 (57.3; 86) |
141 (128.5; 185.0)* |
418 (293; 579) */** |
Sclerostin | 229 (220.8; 230) |
260 (230; 308.75) |
163 (148; 248) |
WIF–1 | 154.5 (56.8; 573.8) |
140.0 (124.8; 171.3) |
2805 (1636.8; 3212.5)*/** |
DVL–1 | 110 (97.5; 349.3) |
482.5 (360; 696.75) |
1002.5 (271.3;1204)*/** |
GSK–3α | 277.00 (238.3; 687.50) |
785* (371.0; 1317.50) |
200.00 (180.00; 222.00)*/** |
GSK–3β | 105.00 (102.5; 110.0) |
295.0 (190.0; 695.0) |
105.00 (100.00; 110.00) |
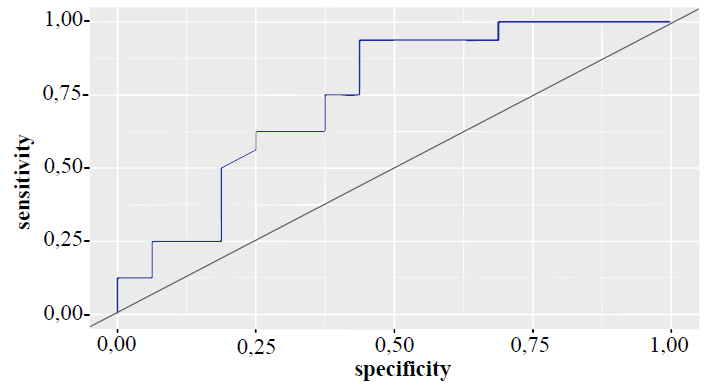
ROC curve of β-catenin levels in patients with MI and hyperlipidemia or without hyperlipidemia.
According to our data, the serum level of sclerostin varied in the patients and was reduced in patients with MI, but there were no significant differences with similar indicators in other groups (p>0.05). However, in 40% of patients with MI, serum sclerostin values were recorded at the lower limit (140–160 pg/ml).
The highest values of sclerostin in the blood serum (290–350 pg/ml) were obtained in patients with CMS and obesity of 2 and 3 degrees (with increasing degrees of obesity, the level of sclerostin increased).
A study of WNT inhibitory factor 1 (WIF-1) as an extracellular antagonist of the WNT signaling pathway [11, 17] showed that its concentration in the blood serum of group III patients was 2805 [1637; 3212] pg/ml, which is on average 18 times higher than in HI and 20 times higher than in CMS (p<0.001). Patients with transmural MI had WIF-1 levels more than 20% lower than patients with non-transmural MI (p=0.172). It is important to pay attention to the results of the correlation between the concentration of WIF-1, the level of leukocytes, and ESR (r = – 0.81, r = – 0.70, p<0.001), as well as the presence of direct correlation between WIF-1 and β-catenin (r = 0.743; p<0.001) in patients with MI. There were no differences of statistical significance in the serum concentrations of WIF-1 in patients of groups I and II.
As can be seen from Table 2, a wide range of variations in DVL–1 values in the blood serum of the studied individuals was obtained. The median value in patients with acute MI was 1002 pg/ml, and the interquartile range was from 271 to 1204 pg/ml. At the same time, an increase of statistical significance in the level of DVL-1 was revealed in patients with MI compared with the indicators of groups I and II.
Patients with Q-positive MI (according to ECG) had maximum DVL-1 values at a level of more than 1500 pg/ml. It was found that patients of groups II and III had the serum level of DVL-1 correlated with cholesterol (p=0.025 and p=0.008) and LDL (p=0.040 and p=0.006). In addition, direct correlations between DVL–1 and the level of β-catenin (p<0.005 and p<0.001), as well as with WIF-1 (r = 0.603, p<0.001) in group III, were established.
Taking into account the important role of Glycogen Synthase Kinase 3 (GSK-3) in the pathophysiology of cardiometabolic diseases [18, 19], serum levels of GSK-3α and GSK-3β were assessed. Intergroup differences of statistical significance were revealed among patients of groups I, II, and III (Table 2, p<0.001). Patients with MI had a great decrease in the content of GSK-3α in the blood serum, and patients with cardiometabolic diseases had an increase. The highest levels of GSK-3α were revealed in atherosclerosis (above 1200 pg/ml) and obesity of grade 3 (with body mass index of 40–44.9 kg/m2). According to our studies, GSK-3β levels in the blood serum in patients of groups II and III did not have statistically significant differences from HI. There were also no reliably significant correlations with clinical and laboratory parameters (Table 1).
3.4. Results of JAK/STAT Protein Analysis
The involvement of the JAK/STAT system in molecular trans-signaling in various pathological conditions [20, 21] led to studies of proteins STAT-1, STAT-3, and STAT-6 in cardiometabolic diseases and acute MI. We measured the concentration of these circulating proteins using ELISA.
The results obtained are presented in Table 3.
In accordance with the bibliographic data on the possible participation of STAT-1 in adipogenesis and pathogenesis of CHD [22-24], studying the features of its production was of great interest. A significant decrease in the serum concentration of STAT-1 as the pathological process progressed compared to HI was observed. In patients of groups II and III, correlations between STAT-1 levels and lipid profile parameters were established. The most significant were relationships with triglycerides (r = – 0.441, p = 0.019 and r = – 0.505, p = 0.003).
Parameter, pg/ml | Group I (n = 50) | Group II (n = 38) | Group III (n = 40) |
---|---|---|---|
STAT-1 | 600.5 (440.5; 1075.5) |
410.7 (0.3; 0.55)* |
370 (245.3; 422.8)* |
STAT-3 | 589.8 (285.1; 1370.0) |
390 (0.21; 0.64)* |
270 (0.15; 0.63)* |
STAT-6 | 558.8 (241.3; 645.1) |
541.1 (222.7; 1510.0) |
184.3 (151.7; 647.7)*/** |
Indicators | Characteristic of Correlation | |||||
---|---|---|---|---|---|---|
Group II (n = 38) | Group III (n = 40) | |||||
r | Correlation Strength on the Chaddock scale |
p | r | Correlation Strength on the Chaddock scale |
p | |
STAT-1 – BMI | –0.667 | Noticeable | <0.001* | –0.483 | Moderate | 0.005* |
STAT-3 – BMI | 0.353 | Moderate | 0.065 | 0.372 | Moderate | 0.036* |
STAT-6 – BMI | –0.226 | Weak | 0.247 | –0.536 | Noticeable | 0.002* |
Despite the large number of studies of STAT-3 in metabolic disorders and cardiovascular pathology [20, 22, 23], there are only a few studies assessing serum levels of STAT-3 in patients. Our analysis established that in the studied groups of patients, the level of STAT-3 protein in the blood serum was significantly lower than in the control group. There were no intergroup differences in STAT-3 values in patients of groups II and III. Statistically significant close correlations were established for STAT-3 with LDL and VLDL (r = 0.512, p = 0.005 and r = 0.513, p = 0.005) only in patients with CMS.
Considering the documented role of STAT-6 in the genesis of inflammation, the data obtained on a great decrease in the level of this protein in patients with MI are important, with minimal threshold values observed in 50% of patients in group III (125–150 pg/ml).
Correlation analysis of serum STAT-6 levels with laboratory test results revealed a negative correlation between STAT-6 and LDL (r = – 0.555, p = 0.002) in patients with CMS. Intergroup differences in STAT-6 values were typical for patients in groups I and III, groups II and III (p<0.005).
More than that, the data obtained on the correlation between STATs proteins and BMI is demonstrated in Table 4.
4. DISCUSSION
Despite the significant progress achieved in recent years in the diagnosis and treatment of CVD, patients with CMS, who have a high risk of developing myocardial infarction and acute forms of coronary artery disease, still remain one of the main problems of modern healthcare [1-4].
Recent research on studying the pathophysiology of cardiometabolic diseases proved the importance of the WNT and JAK/STAT signaling pathways [5-9, 20, 21, 23, 25].
Particular attention is paid to the study of WNT–signaling, associated with many immunobiological processes both in the embryonic and postnatal periods, responsible for differentiation, proliferation, and migration of cells [9, 11, 26], and also plays an important role in the processes of angio- and atherogenesis [10, 15, 16]. All three known WNT signal transduction pathways (canonical WNT/β-catenin, non-canonical WNT/Ca2+, and planar cell polarity pathway) are involved in the pathogenesis of cardiometabolic diseases.
Since WNT signaling plays an important role in the embryonic development of the heart and blood vessels, many components of this pathway are reexpressed in cardiovascular pathology in the postnatal period [9-11, 25]. Accumulating evidence describes a critical role for WNT in multiple pathophysiological mechanisms in the development and progression of atherosclerosis, ranging from endothelial dysfunction with lipid deposition to the inflammatory response followed by atheromatous plaque formation [9-11, 25].
It is proved that reactivation of the canonical WNT pathway after acute heart attack negatively affects myocardial healing, leading to cardiomyocyte death and fibrosis development [9, 14].
However, the complex interaction of WNT signaling with other molecular pathways makes it difficult to formulate a unified pathogenetic concept of the development of cardiometabolic diseases, and this deserves further study.
Recently, the JAK/STAT inflammatory pathway has attracted interest, the dysregulation of which is associated with various CVDs [12, 13]. Activation of Janus-kinases (JAKs) and their downstream proteins, Signal Transducers, and Activators of transcription (STATs) is necessary for the transmission of signals of growth factors and cytokines, which play an important role in the physiology and pathophysiology of the heart. STATs regulate the expression of genes encoding proteins involved in inflammation, molecular trans-signaling, apoptosis, angiogenesis, adipogenesis, etc [21, 27, 28]. All seven members of the STAT family are known to be expressed in the heart and cultured cardiomyocytes, fibroblasts, and endothelial cells [29, 30]. At the same time, most of the available information on the role of JAK/STAT in cardiometabolic diseases relates to STAT-1 and STAT-3.
However, most of the data published today indicating the activation of WNT and JAK/STAT signaling are established in experimental animal models or based on genetic studies, and only a few studies used the quantification of individual protein components of WNT and JAK/STAT - signaling pathways in patients [13, 21].
In this study, we assessed the production features of some JAK/STAT proteins (STAT-1, STAT-3, STAT-6) and WNT (β-catenin, sclerostin, WIF-1, GSK-3α, GSK-3β, DVL-1) signaling of patients with cardiometabolic pathology: patients with acute MI and patients with CMS who are at high risk of developing MI.
The results of studies of β-catenin in the blood serum showed a wide variability in its values in patients of all groups. A significant increase in the concentration of β-catenin was revealed in patients of group III, compared with HI, which is consistent with the bibliographic data about increased canonical WNT signaling and an increase in the level of β-catenin, which is involved in the control of the proliferative ability of cardiomyocytes, promotes the activation of stem cells, necessary for the restoration of the myocardium after damage [31]. In addition, we observed an increase in the concentration of β-catenin in patients of group II, which was most pronounced with BMI>35 kg/m2, which confirms the results of experimental studies on the increased expression of β-catenin in the adipose tissue of obese patients [32].
We found a significant increase in the level of lipid metabolism parameters (TG, cholesterol, LDL, VLDL) in all patients against the background of increased concentration of β-catenin in the blood serum, as well as a high positive correlation between β-catenin and VLDL in patients of group II, which indicate the connection between impaired lipid profile and WNT-morphogenic proteins and are consistent with literature data [33].
A trend towards a decrease in the level of sclerostin, as a potential participant in extraosseous calcification and one of the antagonists of the WNT signaling [34, 35], was identified in patients with MI (in 40% of patients; sclerostin values were recorded at the level of the lower limit). It may be associated with the excessive demand and expense for the implementation of the pathophysiological process.
Our established increase in sclerostin levels as the degree of obesity increases in patients with CMS is consistent with the work of Anouar Aznou, Rick Meijer et al. [36] and may indicate remodeling of adipose tissue.
It is known that WNT Inhibitory Factor-1 (WIF-1) is an extracellular antagonist of the WNT signaling pathway and reflects altered WNT signaling in early atherogenesis, being an important predictor of future adverse cardiovascular events [17].
In the study, we found that the serum concentration of WIF-1 increased only in participants in group III. Considering the data on the relationship between WIF-1 and the degree of activity of the inflammatory response [37, 38], the results of the correlation of statistical significance between the concentrations of WIF-1, WBC, and ESR are noteworthy. The revealed presence of a direct correlation between WIF-1 and β-catenin shows aberrant WNT/β-catenin signal transmission during the MI development.
Currently, there are only a few studies describing the role of DVL-1 (Dishevelled) in CVD, participating in both canonical and non-canonical transmission of WNT signals [39]. Analysis of the results revealed that patients with acute MI have DVL-1 levels significantly higher than groups I and II. Patients with transmural MI have maximum DVL-1 (more than 1500 pg/ml), which may prove the bibliography on the peak of DVL-1 expression in the first 7 days of acute MI [40]. Significant correlations were noted between DVL-1, cholesterol, and LDL, which confirms the role of DVL-1 as a molecular mediator combining WNT signaling and hyperlipidemia [41].
We found the direct correlation of DVL-1 with β-catenin, which indicates a possible compensatory overproduction of DVL-1, necessary for the degradation of β-catenin, the level of which, as we have already noted before, was increased in patients with MI [42]. In addition, data were obtained on a statistically significant correlation noted between DVL-1 and WIF-1 (in patients of group III), which in turn confirms the significant role of DVL-1 as one of the main integrators of canonical and noncanonical transmission of WNT–signals.
Currently, two isoforms of GSK-3 (GSK-3α and β) are described, which have a wide range of activities, from the regulation of cellular metabolism to the control of cell growth and differentiation. Dysregulation of GSK-3 is described in various diseases, including cardiovascular pathology and metabolic disorders. Experimental studies examining GSK-3 in the pathogenesis of CVD confirmed the key role of GSK-3 signaling in the proliferation of cardiomyocytes and identified GSK-3β as a negative regulator of their hypertrophic response, and also identified its role in the regulation of metabolic processes [18, 19, 43, 44].
We did not get statistical differences in patients of all groups while studying the GSK-3β content in blood serum. It may be due to the dysregulation of other signaling pathways in which GSK-3 is involved.
At the same time, statistically significant intergroup differences in GSK-3α levels were established among patients of all groups. Patients with MI had a significant decrease in serum concentration GSK-3α, and patients with cardiometabolic diseases had an increase. Patients with atherosclerosis and obesity of grade 3 had the highest levels of GSK-3α which proves the proatherogenic role of GSK-3α and its participation in the process of adipogenesis [44].
Recognition in recent decades of the inflammatory genesis of atherosclerosis and CVD has led to the interest of researchers in studying the JAK/STAT pathway in the modulation of cardiovascular risk [12, 13, 20, 21].
We revealed a proof decrease in the blood level of STAT-1 as the pathological process progressed compared to HI, which may be associated with the severity of inflammation, the regulation of which is carried out with the participation of STAT-1, which provides the effects of a number of inflammatory cytokines (IL-6, IFN-α, IFN–ɣ, etc.) [12, 13, 21].
At the same time, the selectivity of STAT-1 involvement by cytokines and its participation in the implementation of cytokine effects in the pathophysiology of cardiovascular inflammation requires further study.
Unlike the pro-inflammatory STAT-1, STAT-3 has both pro- and anti-inflammatory characteristics [13, 21, 22]. However, despite the growing amount of knowledge about the function of STAT-3 in various inflammatory diseases [12, 13], patterns of dysregulation of JAK/STAT-3 signaling associated with metabolic dysfunction, pro- and antiatherogenic effects of STAT-3 in cardiovascular pathology are still a matter of debate and need clarification.
Our analysis established that in the studied groups of patients, the level of STAT -3 protein in the blood serum was significantly lower than in the control group, which may be due to the increased demand for STAT-3 for its cardioprotective effect.
The least studied component of the JAK/STAT signaling pathway is STAT-6, which demonstrates profibrotic activity and is involved in inflammatory diseases of various origins [45, 46]. In this regard, the data obtained in our study on a significant decrease in the serum concentration of STAT-6 in patients with MI is noteworthy, which is likely due to its participation in the implementation of inflammation during an acute ischemic process in the myocardium.
In addition, the established correlations between STAT-1, STAT-3, and STAT-6 levels and BMI and lipid profile of patients in groups II and III confirm the participation of activated STATs in adipogenesis and the regulation of lipid metabolism [13, 28, 47].
Therefore, the data presented on changes in the production of proteins of the WNT and JAK/STAT signaling expand our understanding of cardiometabolic disease immunopathogenesis. As outstanding modulators of inflammation, JAK/STAT and WNT signaling proteins represent interesting therapeutic targets, targeted modulation of aberrant production may become a new strategy for the treatment of CVD. Besides, expanding the panel of detectable molecular biomarkers will improve prognostic stratification and early identification of patients at high cardiovascular risk, as well as predicting adverse outcomes.
Some limitations of our study should be noted. First, our study included a small cohort of patients, and we plan to increase their number as we continue our research on this topic. Secondly, we studied the features of serum production of only some WNT and JAK/STAT proteins, which is insufficient to form a complete understanding of the interaction of these signaling pathways.
CONCLUSION
The study of WNT and JAK/STAT regulatory cascades opens new horizons in the immunopathophysiology of cardiometabolic diseases. Moreover, modern concepts linking cardiovascular and metabolic diseases with altered immune reactivity substantiate the relevance of developing complex diagnostic approaches using various biomarkers of WNT and JAK/STAT signaling in modern multimarker models to assess the likelihood of adverse cardiovascular events and promise to lead to the creation of new treatment methods and the development of new areas of cardioimmunology.
AUTHORS’ CONTRIBUTIONS
P.M.: contributed to the study’s concept and design; K.A.: collected the data; S.I. wrote the paper
All authors reviewed the results and approved the final version of the manuscript.
LIST OF ABBREVIATIONS
ALP | = Alkaline phosphatase |
ALT | = Alanine aminotransferase |
AMI | = Acute myocardial infarction |
AST | = Aspartate aminotransferase |
BMI | = Body mass index |
CMS | = Cardiometabolic syndrome |
CVD | = Cardiovascular diseases |
DBP | = Diastolic blood pressure |
ECG | = Electrocardiogram |
ELISA | = Enzyme-linked immunosorbent assay |
ESR | = Erythrocyte sedimentation rate |
HDL | = High density lipoproteins |
HI | = Healthy individuals |
IHD (CHD) | = Ischemic (coronary) heart disease |
RBC | = Red blood cells |
WBC | = White blood cells |
ETHICAL STATEMENT
The study was conducted in accordance with Goоd Clinical Practice standards and the principles of the Helsinki Declaration, the Ethics Committee of the Orel State University, Russia named after I.S. Turgenev (Protocol No.17 of December 10, 2019).
CONSENT FOR PUBLICATION
All patients signed an informed consent form for the use of their examination results for scientific purposes.
AVAILABILITY OF DATA AND MATERIAL
The data supporting the findings of the article cannot be made available due to ethical, legal, or commercial restrictions, as the study was financially supported by the Ministry of Science and Higher Education of Russian Federation.
FUNDING
This study was financially supported by the Ministry of Science and Higher Education of Russian Federation at the Orel State University, Russia named after I.S. Turgenev during implementation of the project as part of a government assignment No. 075-00196-24-02 for 2024 and for the planning period 2025 and 2026 dated 04/01/2024, project No. FSGN-2024-0007 (1023110800218-7-3.2.4;3.1.3;2.6.1).